The beauty of this technology lies in its simplicity and efficiency. Instead of crafting entirely new nanoparticles for each specific application, researchers can now employ a modular nanoparticle base and conveniently attach proteins targeting a desired biological entity. In the past, creating distinct nanoparticles for different biological targets required going through a different synthetic process from start to finish each time. But with this new technique, the same modular nanoparticle base can be easily modified to create a whole set of specialized nanoparticles.
“This is a plug and play platform technology that allows for rapid modification of a functional biological nanoparticle,” said Liangfang Zhang, a professor of nanoengineering at the UC San Diego Jacobs School of Engineering.
Zhang and his team detail their work in a paper published Oct. 30 in Nature Nanotechnology.
The modular nanoparticles consist of biodegradable polymer cores coated with genetically modified cell membranes. The key to their modular design is a pair of synthetic proteins, known as SpyCatcher and SpyTag, that are specifically designed to spontaneously—and exclusively—bind with each other. This pair is commonly used in biological research to combine various proteins. In this study, Zhang and his team harnessed the pair to create a system for attaching proteins of interest to a nanoparticle surface with ease.
Here’s how it works: SpyCatcher is embedded onto the nanoparticle surface, while SpyTag is chemically linked to a protein of interest, such as one targeting tumors or viruses. When SpyTag-linked proteins come into contact with SpyCatcher-decorated nanoparticles, they readily bind to each other, enabling proteins of interest to be effortlessly attached to the nanoparticle surface. For example, to target tumors, SpyTag can be linked to a protein designed to seek out tumor cells, and that SpyTag-linked protein is then attached to the nanoparticle. If the target shifts to a specific virus, the process is similarly straightforward: simply link SpyTag to a protein targeting the virus and attach it to the nanoparticle surface.
“It’s a very simple, streamlined and straightforward approach to functionalizing nanoparticles for any biological application,” said Zhang.
To create the modular nanoparticles, the researchers first genetically engineered human embryonic kidney (HEK) 293 cells—a commonly used cell line in biological research—to express SpyCatcher proteins on their surface. The cell membranes were then isolated, broken into smaller pieces, and coated onto biodegradable polymer nanoparticles.
These nanoparticles were subsequently mixed with SpyTag-linked proteins. In this study, the researchers used two different proteins: one targeting the epidermal growth factor receptor (EGFR) and the other targeting human epidermal growth factor receptor 2 (HER2), both of which are prevalent on the surface of various cancer cells.
As a proof of concept, the researchers tested these nanoparticles in mice with ovarian tumors. The nanoparticles were loaded with docetaxel, a chemotherapy medication, and administered to mice via intravenous injection every three days for a total of four injections. Treatment with these nanoparticles suppressed tumor growth while improving survival rate. Treated mice had median survival of 63 to 71 days, while the median survival of untreated mice was 24 to 29 days.
The researchers are looking to further improve the modular nanoparticle platform for targeted drug delivery.
In addition to cancer treatment, Zhang is excited about other potential applications of this technology. “Because we have a modular nanoparticle base, we can easily attach a neutralizing agent on the surface to neutralize viruses and biological toxins,” he said. “There is also potential for creating vaccines by attaching an antigen on the nanoparticle surface using this modular platform. This opens the door to a variety of new therapeutic approaches.”
Paper: “A modular approach to enhancing cell membrane-coated nanoparticle functionality using genetic engineering.” Co-authors include Nishta Krishnan*, Yao Jiang*, Jiarong Zhou, Animesh Mohapatra, Fei-Xing Peng, Yaou Duan, Maya Holay, Sanam Chekrui, Zhongyuan Guo, Weiwei Gao and Ronnie H. Fang, UC San Diego.
*These authors contributed equally to this work
This work was supported by the Defense Threat Reduction Agency Joint Science and Technology Office for Chemical and Biological Defense (HDTRA1-21-1-0010), the National Institutes of Health (R01CA200574, R21AI159492, and R21AI175904) and the National Science Foundation (DMR-1904702).
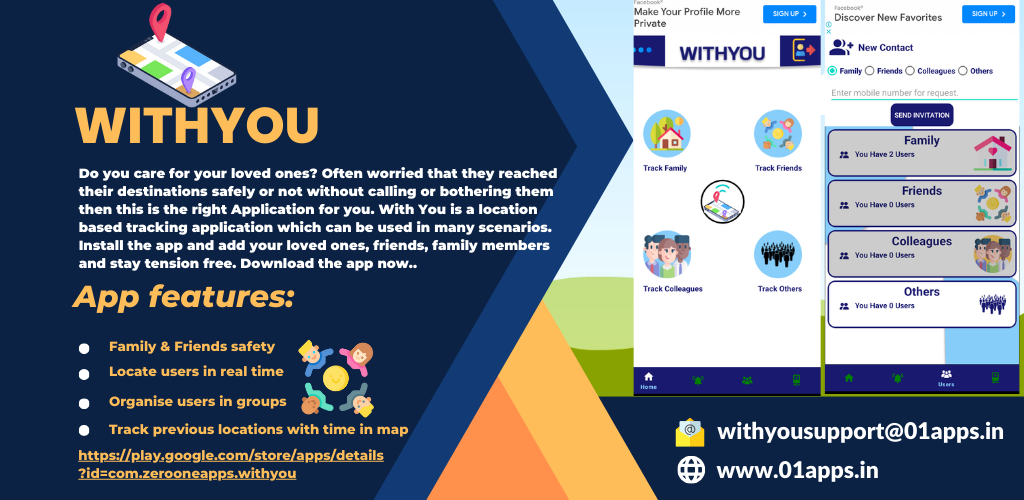