When someone breaks a bone in their lower leg, the fracture can often be complex, making the healing process lengthy and involved. In these cases, it is usual for the fractured bone to be surgically stabilized using an orthopaedic implant. Researchers at Saarland University and the German Research Center for Artificial Intelligence (DFKI) have developed a personalized therapeutic concept that significantly reduces the chances of a patient suffering post-operative complications. The procedure they have developed allows them to create an implant tailored precisely to the patient’s individual fracture. As this personalized fixation plate is designed to accommodate the forces and loading patterns that arise during the patient’s path to recovery, it can significantly improve the fracture healing process. The work of the Saarbrücken team of Stefan Diebels, Michael Roland, Philipp Slusallek and Tim Dahmen, which combines applied mechanics and computational graphics, received funding from the Federal Ministry of Education and Research (BMBF).
While theatrical performers use the phrase ‘break a leg’ to wish each other good luck before going on stage, breaking a leg is really not something any of us want to experience – actor or not. A fracture of the lower leg, which is often the result of a motorcycle, sports or skiing accident, can often prove particularly challenging for the patient. The path to recovery is often difficult and prolonged and can also be extremely expensive. Multiple operations, lengthy treatment regimens and managing infection risks can result in total therapy costs in excess of a hundred thousand euros.
No two fractures are ever the same. They differ in how the bones and bone fragments are twisted, displaced, bent or shortened relative to one another: from oblique fractures, where the fracture line runs diagonal to the long axis of the bone, to spiral fractures, where one of more parts of the fractured bone have been twisted, to comminuted fractures in which the bone is broken into several pieces. ‘Up until now, the medical treatment of fractures has involved the use of standard implants,’ explains Stefan Diebels, Professor of Applied Mechanics at Saarland University. A standard-sized orthopaedic plate is fixed to the fractured bone with screws to allow the bone to heal. The surgeon uses his or her experience to decide on how many screws to use and where they should be placed.
A new therapeutic approach that is adapted to each individual fracture can improve the bone healing process and can also prevent complications. The procedure has been developed by Professor Diebels and Dr. Michael Roland working together with the computer scientists Professor Philipp Slusallek and Dr. Tim Dahmen at Saarland University and DFKI. The project involved fruitful collaboration with medical scientists at Witten/Herdecke University and with industrial partners.
By exploiting their expertise in engineering mechanics, computer science and image processing, the researchers are able to precisely map out and simulate each fracture. The ability to biomechanically analyse the fracture means that the researchers can predict precisely where the greatest load will be experienced by the bone and the fixation plate during different motions, such as walking, sitting down or climbing stairs. By knowing how best to stabilize the bone, they are able to determine the ideal shape and structure of the implant and how big the fracture gap needs to be to optimize healing. Bespoke implants can therefore be manufactured for each patient, tailored to meet the specific characteristics of the fracture. All stages of the fabrication process, from the automatic analysis of clinical computed tomography data to the 3D-printing of the final orthopaedic implant, have been optimized so that the patient can be operated on after only a few days, once the swelling around the leg fracture has subsided. Fractures that are treated in this way heal more quickly and with lower risk of non-union.
The team studied the forces that act in and on a broken shin bone (tibia) and they identified the local loading patterns of the bone and the implant during typical movement situations, such as walking around a bend, walking up steps, sitting down or jumping. These loading patterns have a substantial influence on how a broken bone actually heals. ‘Knowing how loads will be distributed in an individual fracture and which forces will be active, enables us to determine the precise shape of the implant so that it matches the specific geometry of the fracture and provides optimal support for the healing process. It even allows us to calculate how many screws will be needed and where they should be located,’ explains Stefan Diebels. Surgeons often insert extra screws so that they can be sure that the fixation plate really will hold the fractured bone together. By knowing more about the fracture biomechanics, surgeons will be able to work more precisely.
‘Medical practitioners can use our procedure to evaluate the best treatment options,’ explains AI expert Professor Philipp Slusallek. He and his research team use imaging techniques and simulations to visualize loading patterns to make the data simpler to interpret. They analysed a large number of computed tomography datasets of real fractures and used this data to teach the computer to distinguish between tissue, air, metal and image artefacts – just like an experienced surgeon would when studying a CT image. The engineering team in Saarbrücken carried out a large number of experiments and gait analyses in order to acquire loading data on bones. The bones were broken, the fractures stabilized with implants and the bone and implant then studied in a test rig to determine the loads acting in different movement scenarios. ‘To be able to calculate the stresses and strains arising in the plate implant and in the bone structure, we have developed simulation techniques that involve the efficient implementation of well-established finite element methods on modern computer hardware coupled with novel machine learning algorithms,’ says computer scientist Dr. Tim Dahmen. The data from the engineering team was used to train neural networks, which in turn fed into 3D mathematical models that were capable of visualizing the load distributions in and around the fracture site.
The team was particularly interested in learning what was happening in the fracture gap between the bone fragments when the fracture underwent loading. In order for a fractured bone to heal, the bone needs to experience a certain level of loading as this provides the mechanical stimulus required for the bone growth. Too much loading, however, can be detrimental to the healing process and can lead to further damage. ‘The fracture gap has to allow micromovements to occur. So the implant must be designed to permit just the right amount of motion,’ says Tim Dahmen. The simulations also make use of artificial intelligence techniques that enable rapid and automated evaluation of the 3D models. Fractures and implants can now be analysed individually to determine their ability to withstand loading at critical points, which enables an ideally shaped implant to be configured and then 3D-printed.
The project ‘IIP-Extreme – Optimized Patient-Specific Implants’ received a total of around 2.5 million euros in funding from the German Federal Ministry of Education and Research.
At the end of 2019, the researchers Stefan Diebels, Philipp Slusallek and Tim Dahmen together with trauma surgeon Tim Pohlemann from the Faculty of Medicine at Saarland University and the smart materials expert Stefan Seelecke, began working on a follow-up project that has received eight million euros in funding from the Werner Siemens Foundation: The goal of the new project is to develop a smart implant that can monitor the healing process, warn when the implant is being incorrectly loaded and actively mechanically adapt itself to ensure that fractured bone is given the best chance of healing properly.
###
A press release on the follow-up project is available (in German) here:
https:/
/
www.
uni-saarland.
de/
universitaet/
aktuell/
artikel/
nr/
21365.
html
Background:
The project ‘IIP-Extreme – Optimized Patient-Specific Implants’, which received 2.5 million euros in funding from the Federal Ministry of Education and Research, was a collaborative project involving researchers from Saarland University and the German Research Center for Artificial Intelligence (DFKI) as well as Professor Bertil Bouillon and Dr. Thorsten Tjardes from the Department of Orthopaedics and Trauma Surgery at Witten/Herdecke University. Other project partners were Otto Bock Healthcare GmbH from Duderstadt and Karl Leibinger Medizintechnik GmbH & Co. KG from Mühlheim/Donau.
The follow-up project ‘Smart Implants’, which has been awarded eight million euros in funding from the Werner Siemens Foundation and is headed by emergency surgeon Professor Tim Pohlemann, is also an interdisciplinary collaborative project involving Professor Stefan Diebels and his team at the Applied Mechanics Lab, computer scientists Professor Philipp Slusallek and Dr. Tim Dahmen and their team at the German Research Center for Artificial Intelligence(DFKI) and the smart materials specialists led by Professor Stefan Seelecke at Saarland University and the Center for Mechatronics and Automation Technology (ZeMA).
A press release on the follow-up project is available (in German) here:
https:/
/
www.
uni-saarland.
de/
universitaet/
aktuell/
artikel/
nr/
21365.
html
Press photographs are available at
https:/
/
www.
uni-saarland.
de/
universitaet/
aktuell/
artikel/
nr/
21884.
html
and can be used free of charge. Please read and comply with the conditions of use.
Contact:
Professor Dr.-Ing. Stefan Diebels:
Tel.: +49 (0)681 302-3958, -2887; Email: s.diebels(at)mx.uni-saarland.de
Professor Philipp Slusallek:
Tel.: +49 (0)681 85775-5377; Email:
[email protected]
Dr. Tim Dahmen:
Tel.: +49 (0)681 85775-1045; Email:
[email protected]
Dr. Michael Roland
Tel.: +49 (0)681 302-3789; Email:
[email protected]
This part of information is sourced from https://www.eurekalert.org/pub_releases/2020-05/su-ama051220.php
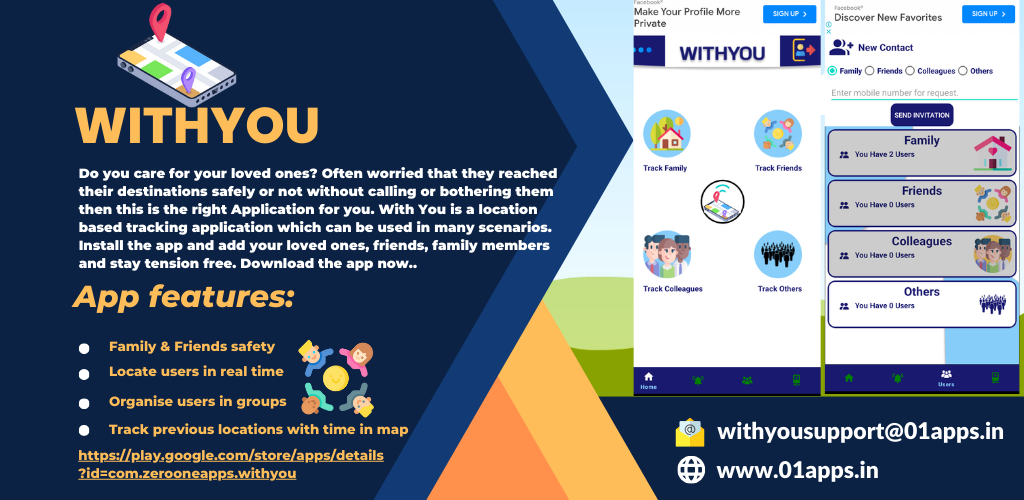