Now a team at Stanford University and the Department of Energy’s SLAC National Accelerator Laboratory has identified exactly which pairs of atoms in a nanoparticle of palladium and platinum – a combination commonly used in converters – are the most active in breaking those gases down.
They also answered a question that has puzzled catalyst researchers: Why do larger catalyst particles sometimes work better than smaller ones, when you’d expect the opposite? The answer has to do with the way the particles change shape during the course of reactions, creating more of those highly active sites.
The results are an important step toward engineering catalysts for better performance in both industrial processes and emissions controls, said Matteo Cargnello, an assistant professor of chemical engineering at Stanford who led the research team. Their report was published June 17 in Proceedings of the National Academy of Sciences.
“The most exciting result of this work was identifying where the catalytic reaction occurs – on which atomic sites you can perform this chemistry that takes a polluting gas and turns it into harmless water and carbon dioxide, which is incredibly important and incredibly difficult to do,” Cargnello said. “Now that we know where the active sites are, we can engineer catalysts that work better and use less expensive ingredients.”
Chemical workhorses
Catalysts are required to perform chemical reactions that would otherwise not happen, such as converting polluting gases from automotive exhaust into clean compounds that can be released into the environment.
In a car’s catalytic converter, nanoparticles of precious metals like palladium and platinum are attached to a ceramic surface. As emission gases flow by, atoms on the surface of the nanoparticles latch onto passing gas molecules and encourage them to react with oxygen to form water, carbon dioxide and other less harmful chemicals. A single particle catalyzes billions of reactions before becoming exhausted.
Today’s catalytic converters are designed to work best at high temperatures, Cargnello said, which is why most harmful exhaust emissions come from vehicles that are just starting to warm up. With more engines being designed to work at lower temperatures, there’s a pressing need to identify new catalysts that perform better at those temperatures, as well as in ships and trucks that are unlikely to switch to electric operation any time soon.
But what makes one catalyst more active than another? The answer has been elusive.
In this study, the research team looked at catalyst nanoparticles made of platinum and palladium from two perspectives – theory and experiment – to see if they could identify specific atomic structures on their surface that contribute to higher activity.
Rounder particles with jagged edges
On the theory side, SLAC staff scientist Frank Abild-Pedersen and his research group at the SUNCAT Center for Interface Science and Catalysis created a new approach for modeling how exposure to gases and steam during chemical reactions affects a catalytic nanoparticle’s shape and atomic structure. This is computationally very difficult, Abild-Pedersen said, and previous studies had assumed particles existed in a vacuum and never changed.
His group created new and simpler ways to model particles in a more complex, realistic environment. Computations by postdoctoral researchers Tej Choksi and Verena Streibel suggested that as reactions proceed, the eight-sided nanoparticles become rounder, and their flat, facet-like surfaces become a series of jagged little steps.
By creating and testing nanoparticles of different sizes, each with a different ratio of jagged edges to flat surfaces, the team hoped to home in on exactly which structural configuration, and even which atoms, contributed the most to the particles’ catalytic activity.
A little help from water
Angel Yang, a PhD student in Cargnello’s group, made nanoparticles of precisely controlled sizes that each contained an evenly distributed mixture of palladium and platinum atoms. To do this, she had to develop a new method for making the larger particles by seeding them around smaller ones. Yang used X-ray beams from SLAC’s Stanford Synchrotron Radiation Lightsource to confirm the composition of the nanoparticles she made with help from SLAC’s Simon Bare and his team.
Then Yang ran experiments where nanoparticles of different sizes were used to catalyze a reaction that turns propene, one of the most common hydrocarbons present in exhaust, into carbon dioxide and water.
“Water here played a particularly interesting and beneficial role,” she said. “Normally it poisons, or deactivates, catalysts. But here the exposure to water made the particles rounder and opened up more active sites.”
The results confirmed that larger particles were more active and that they became rounder and more jagged during reactions, as the computational studies predicted. The most active particles contained the biggest proportion of one particular atomic configuration – one where two atoms, each surrounded by seven neighboring atoms, form pairs to carry out the reaction steps. It was these “7-7 pairs” that allowed big particles to perform better than smaller ones.
Going forward, Yang said, she hopes to figure out how to seed nanoparticles with much cheaper materials to bring their cost down and reduce the use of rare precious metals.
Interest from industry
The research was funded by BASF Corporation, a leading manufacturer of emissions control technology, through the California Research Alliance, which coordinates research between BASF scientists and seven West Coast universities, including Stanford.
“This paper is addressing fundamental questions about active sites, with theory and experimental perspectives coming together in a really nice way to explain the experimental phenomena. This has never been done before, and that’s why it’s quite significant,” said Yuejin Li, a senior principal scientist with BASF who participated in the study.
“In the end,” he said, “we want to have a theoretical model that can predict what metal or combination of metals will have even better activity than our current state of the art.”
Stanford Synchrotron Radiation Lightsource is a DOE Office of Science user facility. SUNCAT, which is a partnership between SLAC and the Stanford School of Engineering, also receives support from the DOE Office of Science.
SLAC is a vibrant multiprogram laboratory that explores how the universe works at the biggest, smallest and fastest scales and invents powerful tools used by scientists around the globe. With research spanning particle physics, astrophysics and cosmology, materials, chemistry, bio- and energy sciences and scientific computing, we help solve real-world problems and advance the interests of the nation.
SLAC is operated by Stanford University for the U.S. Department of Energy’s Office of Science. The Office of Science is the single largest supporter of basic research in the physical sciences in the United States and is working to address some of the most pressing challenges of our time.
Original post https://alertarticles.info
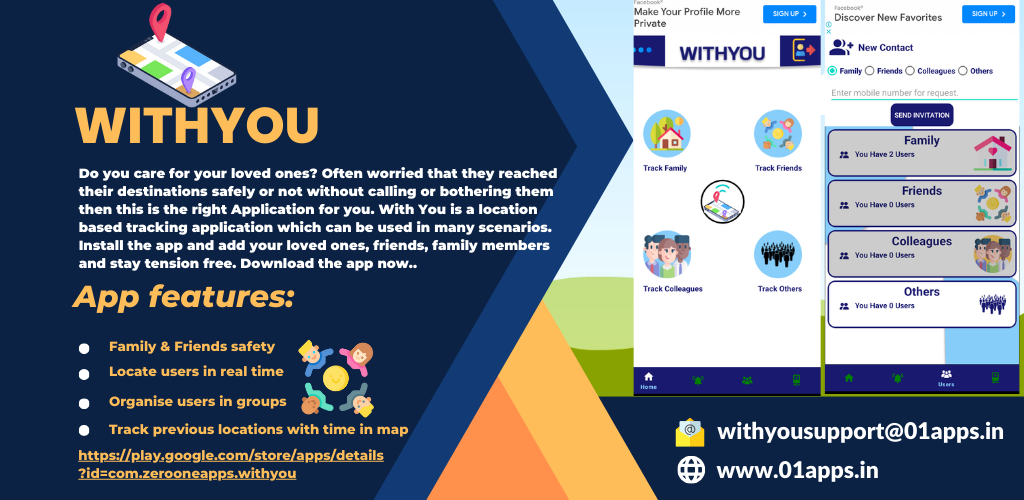