To address this challenge, a team led by researchers at the National Synchrotron Light Source II (NSLS-II) — a U.S. Department of Energy (DOE) Office of Science user facility at DOE’s Brookhaven National Laboratory — used complementary X-ray techniques at two different beamlines to gain new insights into these materials. Their results were published in Physical Review Letters.
New discoveries in a long history
Superconductivity was first discovered in mercury more than 100 years ago. Superconducting materials allow current to flow through them with no resistance and therefore have no power loss. As these materials enter a superconducting state, the persistent electric current allows them to expel a magnetic field and levitate over magnetic materials as well.
Initially, superconducting properties seemed to only appear at extremely low temperatures — -415 degrees Fahrenheit. In the mid-1980s, however, researchers found that copper-based oxide materials, or “cuprates,” can display these properties at -297.7 degrees Fahrenheit. This spearheaded research in “high-temperature” superconductivity and the search for other cuprate-like high-temperature superconductors. If researchers can find a way to engineer materials to superconduct at higher, more practical temperatures, they might one day contribute to eliminating energy losses in the power grid and paving the way for other novel technologies like maglev trains, more efficient MRI machines, and high-capacity energy storage for electric vehicles.
More recently, nickel-based materials have attracted attention as a new family of high temperature superconductors analogous to cuprates. Neodymium nickelate becomes particularly interesting when strontium is added to its structure. This compound is known as an “infinite layer nickelate,” since nickel atoms are arranged in a two-dimensional square lattice that repeats indefinitely in two dimensions, earning the moniker “infinite.”
Superconductivity in nickelates has, so far, only been observed in very thin films. This raises questions about whether the superconducting properties depend on interactions at the interfaces between the nickelate material and its substrate or capping layer. Early studies provided conflicting results on the properties of these materials.
“This system is sensitive to water and oxygen,” explained Jonathan (Johnny) Pelliciari, a beamline scientist at NSLS-II’s Soft Inelastic X-ray Scattering (SIX) beamline, “so past studies used a very thin protective capping layer and attributed electronic orders to the lack of a thick surface layer. Given how sensitive these systems are, small changes or defects can also affect the material’s properties. We wanted to see how much of a role this capping layer was playing and what signals may be spurious.”
To answer this question, the team employed two beamlines at NSLS-II to investigate high quality nickelate thin film samples with and without a capping layer of strontium titanate to see if the layer has an effect on magnetic and electronic properties. Magnetic properties are critical because they relate to the material’s intrinsic electronic structure, which is directly linked to its superconductivity.
Complementary techniques complete the picture
Resonant Elastic X-Ray Scattering (REXS), performed at the Coherent Soft X-ray Scattering (CSX) beamline at NSLS-II, offers researchers a detailed view of a material’s structural properties. This part of the experiment revealed the atomic and electronic structure of the infinite-layer nickelate thin films. Resonant Inelastic X-ray Scattering (RIXS), performed at the SIX beamline, then measured how X-rays lose energy as they scatter off the films. By analyzing the density, motion, and interactions of electrons and spins, researchers gained valuable insight into processes related to electronic and magnetic properties in the material.
Combining these perspectives gave a complete picture of how the material behaved, especially any changes introduced by capping. The group found that the material’s magnetic fluctuations, or “spin excitations,” are present whether or not the capping layer is applied, showing that magnetism is an inherent quality of these nickelates. In capped samples, these magnetic properties are only slightly stronger because of interfacial effects, which might be due to slight structural adjustments at the interface where the capped layer meets the nickelate, crystal defects, or lattice disorder. The data also confirmed that spin excitations in these materials are stable in the superconducting phase, similar to what is seen in cuprates.
“RIXS is very sensitive to magnetism,” said Shiyu Fan, a postdoctoral researcher at SIX and lead author of this study. “Perhaps the most important finding of this research is the evolution of the spin wave in the presence or absence of the capping layer, which points to the magnetic and superconducting properties being intrinsic to the infinite layer nickelate material.”
“The similarity between copper oxide planes in superconducting cuprates and nickel oxide planes in nickelates have had scientists searching for superconductivity in nickelates for 25 years,” said Claudio Mazzoli, lead beamline scientist at CSX. “Now that it has finally been found, we need to understand the differences and commonalities in these two cases and the physics behind them to gain control of this fascinating phenomenon for technological applications.”
The research and the facilities used were funded by the DOE Office of Science.
Brookhaven National Laboratory is supported by the Office of Science of the U.S. Department of Energy. The Office of Science is the single largest supporter of basic research in the physical sciences in the United States and is working to address some of the most pressing challenges of our time. For more information, visit science.energy.gov.
Follow @BrookhavenLab on social media. Find us on Instagram, LinkedIn, X, and Facebook.
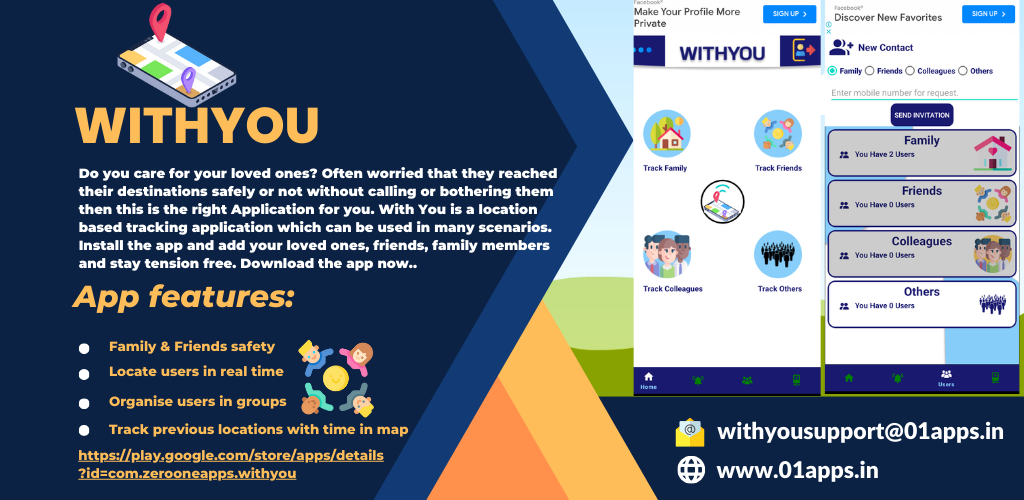