Columbia Engineering researchers report that they developed a new, efficient way to modulate and enhance an important type of nonlinear optical process: optical second harmonic generation—where two input photons are combined in the material to produce one photon with twice the energy—from hexagonal boron nitride through micromechanical rotation and multilayer stacking. The study was published online March 3 by Science Advances.
“Our work is the first to exploit the dynamically tunable symmetry of 2D materials for nonlinear optical applications,” said James Schuck, associate professor of mechanical engineering, who led the study along with James Hone, Wang Fong-Jen Professor of Mechanical Engineering.
A hot topic in the field of 2D materials has been exploring how twisting or rotating one layer relative to another can change the electronic properties of the layered system—something that can’t be done in 3D crystals because the atoms are bond so tightly together in a 3D network. Solving this challenge has led to a new research area termed “twistronics.” In this new study, the team used concepts from twistronics to show that they also apply to optical properties.
“We are calling this new research area ‘twistoptics,’” said Schuck. “Our twistoptics approach demonstrates that we can now achieve giant nonlinear optical responses in very small volumes—just a few atomic layer thicknesses—enabling, for example, entangled photon generation with a much more compact, chip-compatible foot print. Moreover, the response is fully tunable on demand.”
Most of today’s conventional nonlinear optical crystals are made of covalently bonded materials, such as lithium niobate and barium borate. But because they have rigid crystal structures, it is difficult to engineer and control their nonlinear optical properties. For most applications, though, some degree of control over a material’s nonlinear optical properties is essential.
The group found that van der Waals multilayer crystals provide an alternative solution for engineering optical nonlinearity. Thanks to the extremely weak interlayer force, the researchers could easily manipulate relative crystal orientation between neighboring layers by micromechanical rotation. With the ability to control symmetry at the atomic-layer limit, they demonstrated precise tuning and giant enhancement of optical second harmonic generation with micro-rotator devices and superlattice structures, respectively. For the superlattices, the team first used layer rotation to created “twisted” interfaces between layers that yield an extremely strong nonlinear optical response, and then stacked several of these “twisted” interfaces on top of one another.
“We showed that the nonlinear optical signal actually scales with the square of the number of twisted interfaces,” said Kaiyuan Yao, a postdoctoral research fellow in Schuck’s lab and co-lead author of the paper. “So this makes the already large nonlinear response of a single interface orders of magnitude stronger still.”
The group’s findings have several potential applications. Tunable second harmonic generation from micro-rotators could lead to novel on-chip transducers that couple micromechanical motion to sensitive optical signals by turning mechanical motion into light. This is critical for many sensors and devices such as atomic force microscopes.
Stacking multiple boron nitride thin films on top of each other with controlled twist angle demonstrated greatly enhanced nonlinear response. This could offer a new way to manufacture efficient nonlinear optical crystals with atomic precision. These could be used in a broad range of laser (such as the green laser pointers), optical spectroscopy, imaging, and metrology systems. And perhaps most significantly, they could provide a compact means for generating entangled photons and single photons for next-generation optical quantum information processing and computing.
This work was a collaboration carried out at the Energy Frontier Research Center on Programmable Quantum Materials at Columbia, with theory collaborators at Max Planck Institute for the Structure and Dynamics of Matter. The device fabrication was partially done in the cleanroom of the Columbia Nano Initiative.
“We hope,” Schuck said, “that this demonstration provides a new twist in the ongoing narrative aimed at harnessing and controlling the properties of materials.”
About the Study
The study is titled “Enhanced tunable second harmonic generation from twistable interfaces and vertical superlattices in boron nitride homostructures.”
Authors are: Kaiyuan Yao1, Nathan R. Finney1, Jin Zhang2, Samuel L. Moore3, Lede Xian2†, Nicolas Tancogne-Dejean2, Fang Liu4, Jenny Ardelean1, Xinyi Xu1, Dorri Halbertal3, 56334 K. Watanabe , T. Taniguchi , Hector Ochoa , Ana Asenjo-Garcia , Xiaoyang Zhu , D. N. Basov3, Angel Rubio2,7, Cory R. Dean3, James Hone1, P. James Schuck.
1Department of Mechanical Engineering, Columbia University
2Max Planck Institute for the Structure and Dynamics of Matter, Germany
3Department of Physics, Columbia University
4Department of Chemistry, Columbia University
5Research Center for Functional Materials, National Institute for Materials Science, Japan
6International Center for Materials Nanoarchitectonics, National Institute for Materials Science, Japan
7Center for Computational Quantum Physics, Simons Foundation Flatiron Institute
The study was supported mainly as part of Programmable Quantum Materials, an Energy Frontier Research Center funded by the U.S. Department of Energy (DOE), Office of Science, Basic Energy Sciences (BES), under award DE-SC0019443. Theory support involving DFT calculations for this project is supported by the European Research Council (ERC-2015-AdG694097), the Cluster of Excellence AIM, and the Max Planck Institute–New York City Center for Non-Equilibrium Quantum Phenomena (A.R.). The Flatiron Institute is a division AQ7 of the Simons Foundation. N.F. acknowledges support from the Stewardship Science Graduate Fellowship program provided under cooperative agreement number DE-NA0003864. K.W. and T.T. acknowledge support from the Elemental Strategy Initiative conducted by the MEXT, Japan (grant number JPMXP0112101001), JSPS KAKENHI (grant number JP20H00354), and the CREST (JPMJCR15F3), JST. J.Z. acknowledges funding received from the European Union Horizon 2020 research and innovation program under the Marie Sklodowska-Curie Grant Agreement 886291 (PeSD-NeSL).
F.L., X.Y.Z., and J.H. are inventors on a patent application related to this work filed by Columbia University (no. 62/944,753 and 62/963,839, filed 6 December 2019). The authors declare that they have no other competing interests.
###
LINKS:
Paper: https://advances.sciencemag.org/content/7/10/eabe8691.full
DOI: 10.1126/sciadv.abe8691
https://advances.sciencemag.org/
http://engineering.columbia.edu/
https://www.engineering.columbia.edu/faculty/p-james-schuck
https://www.me.columbia.edu/
https://www.engineering.columbia.edu/faculty/james-hone
https://quantum-materials.columbia.edu/
https://www.mpsd.mpg.de/en
http://cni.columbia.edu/
###
Columbia Engineering
Columbia Engineering, based in New York City, is one of the top engineering schools in the U.S. and one of the oldest in the nation. Also known as The Fu Foundation School of Engineering and Applied Science, the School expands knowledge and advances technology through the pioneering research of its more than 220 faculty, while educating undergraduate and graduate students in a collaborative environment to become leaders informed by a firm foundation in engineering. The School’s faculty are at the center of the University’s cross-disciplinary research, contributing to the Data Science Institute, Earth Institute, Zuckerman Mind Brain Behavior Institute, Precision Medicine Initiative, and the Columbia Nano Initiative. Guided by its strategic vision, “Columbia Engineering for Humanity,” the School aims to translate ideas into innovations that foster a sustainable, healthy, secure, connected, and creative humanity.
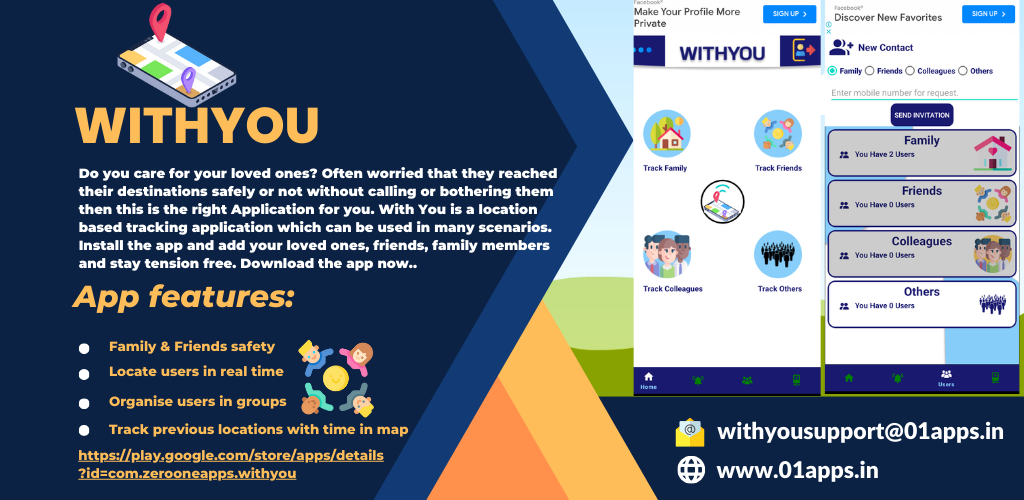