Tarantulas and humans would appear to have nothing in common. But our muscles tell a different story.
Both human and spider muscles contain myosin, a family of motor proteins essential to movement. Scientists don’t have a complete understanding of how myosin triggers muscle movement. But because the myosin in tarantulas is easier to analyze within a lab, researchers have an opportunity to learn more.
“With resources like the APS, we can gain the highly-detailed structural information we need to fully understand fundamental behaviors such as muscle movement.” — Thomas Irving, Illinois Institute of Technology professor and co-author of the study
A research team led by structural biologist Raúl Padrón, a professor at the University of Massachusetts Medical School, has gained powerful insights using X-rays at the Advanced Photon Source (APS), a U.S. Department of Energy (DOE) Office of Science User Facility at DOE’s Argonne National Laboratory. The researchers’ experiments, published in PNAS, revealed in fine detail the structure and behavior of myosin when its switched on and off in tarantula muscles.
Their insights can help scientists better understand the range of ways muscles can behave and the structural basis for human diseases such as hypertrophic cardiomyopathy (HCM), a condition in which human heart muscles contract with too much force.
Myosin’s role in muscle behavior
Muscles normally contract when myosin interacts with another protein called actin. Both appear as filaments, or ropelike fibers. Myosin filaments in particular have hundreds of two-headed structures spread along them. When activated, these heads bind to actin, and this motion generates force.
“When our brain sends an electrical signal to our muscles, instructing them to move, what exactly happens to these myosin heads? That’s the question we wanted to answer with this study,” said Padrón.
Padrón and his peers also set out to explain a phenomenon known as posttetanic potentiation (PTP). PTP occurs when muscles generate an unusually strong force following a prolonged period of stimulation.
“We wanted to study the structural basis of PTP because PTP happens in the skeletal muscle of human and other animals,” Padrón said.
X-ray analysis at the APS
To find answers, Padrón and his team conducted their experiment at the Biophysics Collaborative Access Team (BioCAT) beamline, 18-ID, which is funded by the National Institutes of Health and specially designed to study muscles and other biological fibers at the APS.
Researchers analyzed a live tarantula leg with the powerful X-ray beam generated by the APS. Using the diffraction patterns the beam created as it scattered off the musculature, the team could calculate and characterize molecular structures within the muscle.
“The reason we chose tarantula in particular is because their myosin filaments are well-structured, which allows us to get really good high-quality X-ray diffraction patterns,” Padrón said.
Putting together these diffraction patterns like a series of frames in a movie, they could also track how the myosin heads move before, during and after contraction.
“Thirty or 40 years ago, it would have taken days to get a single pattern, but today we can get hundreds of pictures in a second almost in real time. That’s the power of the APS,” said co-author Weikang Ma, a research assistant professor at the Illinois Institute of Technology (IIT) and a BioCAT beamline scientist.
Insights reveal dynamics of the process
The experimental approach — known as time-resolved synchrotron X-ray diffraction — allowed researchers to see how the two heads of myosin interact with each other in significantly greater detail than has been achieved in other muscle systems, according to BioCAT director Thomas Irving, an IIT professor and co-author of the study.
Among other things, they discovered that, in a relaxed state, myosin heads align with each other in a recurring element known as the interacting head motif (IHM). Other studies have proven that the IHM exists in other animals, including humans and mice, but this study is the first to show IHM is present in live muscle.
Researchers also uncovered that, unlike humans, myosin in tarantulas is not primarily activated by mechanical stress, but by phosphorylation (the addition of a phosphate element). After receiving a signal to contract, one myosin head becomes phosphorylated, causing it to be released from the IHM and change position to bind to actin. The release of one head frees the other to become phosphorylated and active. This sequence of events helps explain how contraction and PTP occur.
Myosin activation and the IHM are linked to human diseases such as HCM, the most common of all genetic heart conditions. Studies indicate that the mutations associated with HCM occur in the interactions between the two myosin heads that form the IHM. What scientists have learned about the IHM and regulating myosin within the APS could give them clues for understanding HCM more and designing drugs to treat the impairments produced by HCM on the IHM.
“With resources like the APS, we can gain the highly-detailed structural information we need to truly understand the structure and function of our own muscles,” said Irving.
The paper, titled “The myosin interacting-heads motif present in live tarantula muscle explains tetanic and posttetanic phosphorylation mechanisms” was published in the Proceedings of the National Academy of Sciences in June 2020. Other co-authors include Sebastian Duno-Miranda, Natalia Koubassova, Kyoung Hwan Lee, Antonio Pinto, Lorenzo Alamo, Pura Bolaños, Andrey Tsaturyan and Roger Craig.
This study was funded by the National Institutes of Health.
The Advanced Photon Source is a DOE Office of Science User Facility. BioCAT is a national user facility funded by the National Institutes of Health.
About the Advanced Photon Source
The U. S. Department of Energy Office of Science’s Advanced Photon Source (APS) at Argonne National Laboratory is one of the world’s most productive X-ray light source facilities. The APS provides high-brightness X-ray beams to a diverse community of researchers in materials science, chemistry, condensed matter physics, the life and environmental sciences, and applied research. These X-rays are ideally suited for explorations of materials and biological structures; elemental distribution; chemical, magnetic, electronic states; and a wide range of technologically important engineering systems from batteries to fuel injector sprays, all of which are the foundations of our nation’s economic, technological, and physical well-being. Each year, more than 5,000 researchers use the APS to produce over 2,000 publications detailing impactful discoveries, and solve more vital biological protein structures than users of any other X-ray light source research facility. APS scientists and engineers innovate technology that is at the heart of advancing accelerator and light-source operations. This includes the insertion devices that produce extreme-brightness X-rays prized by researchers, lenses that focus the X-rays down to a few nanometers, instrumentation that maximizes the way the X-rays interact with samples being studied, and software that gathers and manages the massive quantity of data resulting from discovery research at the APS.
This research used resources of the Advanced Photon Source, a U.S. DOE Office of Science User Facility operated for the DOE Office of Science by Argonne National Laboratory under Contract No. DE-AC02–06CH11357.
Argonne National Laboratory seeks solutions to pressing national problems in science and technology. The nation’s first national laboratory, Argonne conducts leading-edge basic and applied scientific research in virtually every scientific discipline. Argonne researchers work closely with researchers from hundreds of companies, universities, and federal, state and municipal agencies to help them solve their specific problems, advance America’s scientific leadership and prepare the nation for a better future. With employees from more than 60 nations, Argonne is managed by UChicago Argonne, LLC for the U.S. Department of Energy’s Office of Science.
The U.S. Department of Energy’s Office of Science is the single largest supporter of basic research in the physical sciences in the United States and is working to address some of the most pressing challenges of our time. For more information, visit https://energy.gov/science.
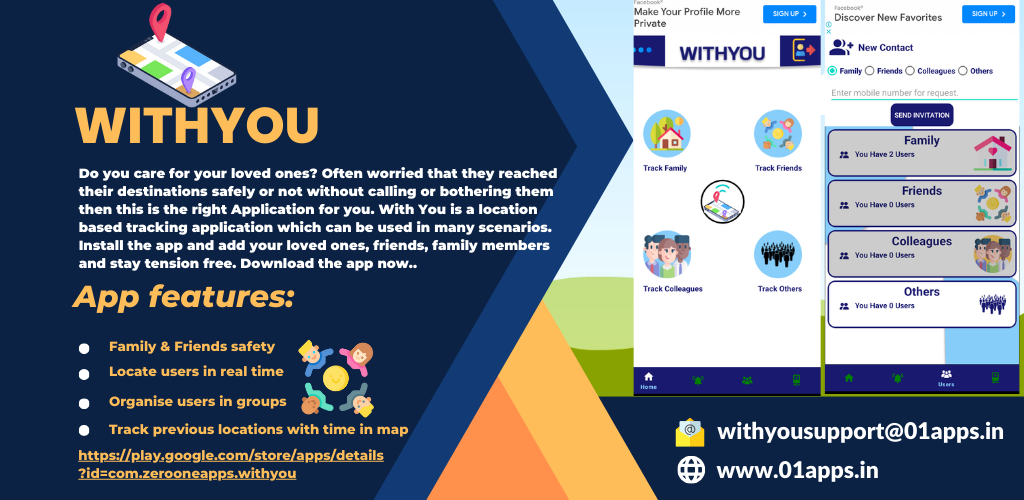