Diana Gamzina, a staff scientist at the Department of Energy’s SLAC National Accelerator Laboratory; Timothy Horn, an assistant professor of mechanical and aerospace engineering at North Carolina State University; and researchers at RadiaBeam Technologies dream of developing the technique to print particle accelerators and vacuum electronic devices for applications in medical imaging and treatment, the electrical grid, satellite communications, defense systems and more.
In fact, the researchers are closer to making this a reality than you might think.
“We’re trying to print a particle accelerator, which is really ambitious,” Gamzina said. “We’ve been developing the process over the past few years, and we can already print particle accelerator components today. The whole point of 3D printing is to make stuff no matter where you are without a lot of infrastructure. So you can print your particle accelerator on a naval ship, in a small university lab or somewhere very remote.”
3D printing can be done with liquids and powders of numerous materials, but there aren’t any well-established processes for 3D printing ultra-high-purity copper and its alloys – the materials Gamzina, Horn and their colleagues want to use. Their research focuses on developing the method.
Indispensable copper
Accelerators boost the energy of particle beams, and vacuum electronic devices are used in amplifiers and generators. Both rely on components that can be easily shaped and conduct heat and electricity extremely well. Copper has all of these qualities and is therefore widely used.
Traditionally, each copper component is machined individually and bonded with others using heat to form complex geometries. This manufacturing technique is incredibly common, but it has its disadvantages.
“Brazing together multiple parts and components takes a great deal of time, precision and care,” Horn said. “And any time you have a joint between two materials, you add a potential failure point. So, there is a need to reduce or eliminate those assembly processes.”
Potential of 3D copper printing
3D printing of copper components could offer a solution.
It works by layering thin sheets of materials on top of one another and slowly building up specific shapes and objects. In Gamzina’s and Horn’s work, the material used is extremely pure copper powder.
The process starts with a 3D design, or “construction manual,” for the object. Controlled by a computer, the printer spreads a few-micron-thick layer of copper powder on a platform. It then moves the platform about 50 microns – half the thickness of a human hair – and spreads a second copper layer on top of the first, heats it with an electron beam to about 2,000 degrees Fahrenheit and welds it with the first layer. This process repeats over and over until the entire object has been built.
The amazing part: no specific tooling, fixtures or molds are needed for the procedure. As a result, 3D printing eliminates design constraints inherent in traditional fabrication processes and allows the construction of objects that are uniquely complex.
“The shape doesn’t really matter for 3D printing,” said SLAC staff scientist Chris Nantista, who designs and tests 3D-printed samples for Gamzina and Horn. “You just program it in, start your system and it can build up almost anything you want. It opens up a new space of potential shapes.”
The team took advantage of that, for example, when building part of a klystron – a specialized vacuum tube that amplifies radiofrequency signals – with internal cooling channels at NCSU. Building it in one piece improved the device’s heat transfer and performance.
Compared to traditional manufacturing, 3D printing is also less time consuming and could translate into cost savings of up to 70%, Gamzina said.
A challenging technique
But printing copper devices has its own challenges, as Horn, who began developing the technique with collaborators from RadiaBeam years ago, knows. One issue is finding the right balance between the thermal and electrical properties and strengths of the printed objects. But the biggest hurdle for manufacturing accelerators and vacuum electronics, though, is that these high-vacuum devices require extremely high quality and pure materials to avoid part failures, such as cracking or vacuum leaks.
The research team tackled these challenges by first improving the material’s surface quality, using finer copper powder and varying the way they fused layers together. However, using finer copper powder led to the next challenge. It allowed more oxygen to attach to the copper powder, increasing the oxide in each layer and making the printed objects less pure.
So, Gamzina and Horn had to find a way to reduce the oxygen content in their copper powders. The method they came up with, which they recently reported in Applied Sciences, relies on hydrogen gas to bind oxygen into water vapor and drive it out of the powder.
Using this method is somewhat surprising, Horn said. In a traditionally manufactured copper object, the formation of water vapor would create high-pressure steam bubbles inside the material, and the material would blister and fail. In the additive process, on the other hand, the water vapor escapes layer by layer, which releases the water vapor more effectively.
Although the technique has shown great promise, the scientists still have a ways to go to reduce the oxygen content enough to print an actual particle accelerator. But they have already succeeded in printing a few components, such as the klystron output cavity with internal cooling channels and a string of coupled cavities that could be used for particle acceleration.
Planning to team up with industry partners
The next phase of the project will be driven by the newly-formed Consortium on the Properties of Additive-Manufactured Copper, which is led by Horn. The consortium currently has four active industry members – Siemens, GE Additive, RadiaBeam and Calabazas Creek Research – with more on the way.
“This would be a nice example of collaboration between an academic institution, a national lab and small and large businesses,” Gamzina said. “It would allow us to figure out this problem together. Our work has already allowed us to go from ‘just imagine, this is crazy’ to ‘we can do it’ in less than two years.”
This work was primarily funded by the Naval Sea Systems Command, as a Small Business Technology Transfer Program with Radiabeam, SLAC, and NCSU. Other SLAC contributors include Chris Pearson, Andy Nguyen, Arianna Gleason, Apurva Mehta, Kevin Stone, Chris Tassone and Johanna Weker. Additional contributions came from Christopher Ledford and Christopher Rock at NCSU and Pedro Frigola, Paul Carriere, Alexander Laurich, James Penney and Matt Heintz at RadiaBeam.
SLAC is a vibrant multiprogram laboratory that explores how the universe works at the biggest, smallest and fastest scales and invents powerful tools used by scientists around the globe. With research spanning particle physics, astrophysics and cosmology, materials, chemistry, bio- and energy sciences and scientific computing, we help solve real-world problems and advance the interests of the nation.
SLAC is operated by Stanford University for the U.S. Department of Energy’s Office of Science. The Office of Science is the single largest supporter of basic research in the physical sciences in the United States and is working to address some of the most pressing challenges of our time.
Original post https://alertarticles.info
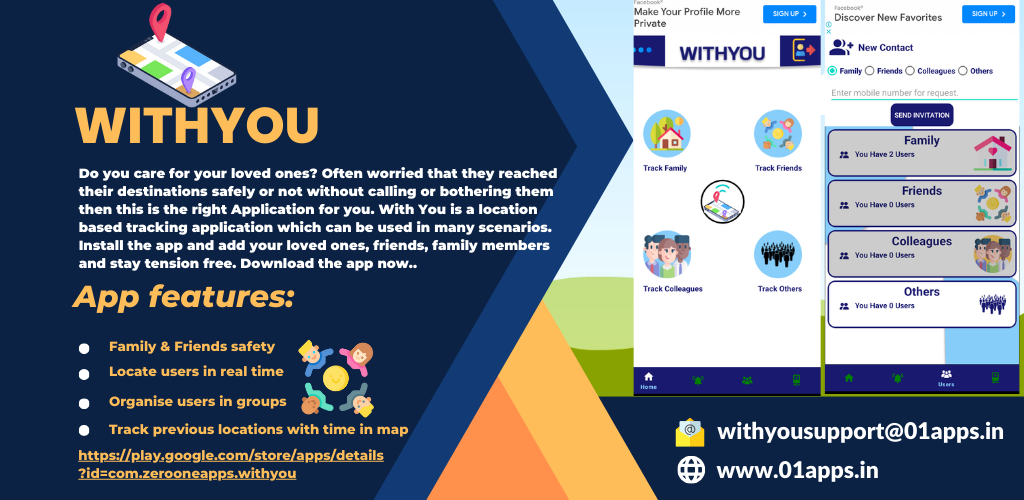