One of the main missions of the U.S. Department of Energy’s Thomas Jefferson National Accelerator Facility is to study the structure of atomic nuclei, which are responsible for most of the mass of ordinary matter. Atomic nuclei are made up of protons and neutrons, which are in turn made up of smaller, point-like particles called quarks and gluons.
Physicists know from previous experiments that what happens at the nuclear scale to protons and neutrons also affects their constituent quarks and gluons – they just don’t know exactly how yet. A novel new framework has tapped on short-range correlations to decipher the particles’ choreography and reveal this connection.
Short-range correlations are quantum mechanical fluctuations that occur between protons and neutrons when they’re close together and strongly interacting. They are an exotic yet universal phenomenon that occur in all nuclei and impact a sizable fraction of nucleons in the nucleus. In results published in Physical Review Letters and also featured in Physics World’s 2024 “Top 10 Breakthroughs of the Year,” a team of nuclear and particle physicists provide evidence that these temporary pairings of protons and neutrons affect the distribution of their constituent quarks and gluons.
“It’s not obvious that these short-range correlations would affect the quarks, but they do,” said Cynthia Keppel, Jefferson Lab’s associate director for experimental nuclear physics and one of the researchers contributing to this study. “You wouldn’t necessarily expect that to happen because quarks are so much smaller than protons and neutrons. But this shows that the nucleus is affecting things at the tiniest levels.”
Studying these correlated pairs will help physicists learn more about the particles that shape our existence.
“This work is a step toward understanding our visible universe,” said Andrew Denniston, a graduate student researcher at the Massachusetts Institute of Technology (MIT) and lead author on the recently published paper. Denniston is a member of Professor Or Hen’s research group at MIT.
“This surprising result significantly enhances our understanding of nuclear binding,” commented Tomas Jezo, a faculty member at the Institute of Theoretical Physics at the University of Münster, and a lead with Michael Klasen of the Münster team, which played an essential role in this international collaborative project.
An interdisciplinary approach
While nuclear and particle physics are often considered as describing different regimes of the universe, inside the nucleus of the atom, they overlap. In this work, nuclear physicists and particle physicists collaborated to develop a novel framework that consistently describes both the particle (quark-gluon) and nuclear (proton-neutron) structure of nuclei, including the complex correlations that exist between the two.
“This work presents a groundbreaking paradigm that establishes a direct link between the nuclear physics description of matter in terms of protons and neutrons and the particle physics schema in terms of quarks and gluons,” said Fredrick Olness, a physics professor at Southern Methodist University. “Bringing together the two communities was a key element in achieving this new insight.”
Olness, a particle physicist, typically studies quarks and gluons. Denniston and his MIT advisor Or Hen, nuclear physicists, study short-range correlations between protons and neutrons.
With input from Klasen, Ježo, Keppel, Olness and other collaborators of this work, Denniston and Hen proposed equations that combine particle and nuclear physics models to separate how quarks and gluons move depending on if they are in short-range correlated proton-neutron pairs or not.
“This work was largely accomplished with the genuine scientific input from young scientists, which is great,” said Keppel on Denniston’s contribution. “It’s a joy to have that kind of collaboration.”
The equation formed the basis of a framework that combines information from nuclear parton distribution functions with information on short-range correlations. Nuclear parton distribution functions describe how much momentum each quark is carrying, while short-range correlations involve the highest-momentum protons and neutrons. A key point about short-range correlations is their universality: they behave the same in all the nuclei they have thus far been measured in.
To test how well this new nuclear PDF framework described real data, the team plugged it into a code developed by the nuclear Coordinated Theoretical-Experimental Project on QCD (nCTEQ). Olness is a founding member of nCTEQ, a multi-institutional collaboration focused on QCD or quantum chromodynamics, the theory of how quarks and gluons interact.
One goal of the collaboration is to study nuclear structures, a project Keppel has tackled as a member of nCTEQ. As the former spokesperson for CTEQ, Keppel had initially suggested the collaboration with Hen’s nuclear research group at MIT.
The nCTEQ code applied the nuclear PDF to about 1,500 data points measuring 19 different nuclei, ranging from hydrogen, which has just one proton, to lead, which has 208 protons and neutrons. The data were collected during a variety of experiments at labs in the U.S. and around the world, including Jefferson Lab.
“Adding the Jefferson Lab data to the nuclear CTEQ fit was critical to this work, as it is the most sensitive to short-range correlations,” said Keppel.
The team was surprised to find that this new model described the data better than the traditional approach.
“Remarkably, the model works exceptionally well, revealing that while some protons and neutrons behave as they do outside the nucleus, others, particularly those in correlated pairs, exhibit drastically different structures,” said Hen. “We showed it before for a very specific case, known as the EMC effect. Now we managed to generalize our approach and use it to consistently explain many different phenomena previously thought to be unrelated”
Getting specific with nuclei
This work also revealed interesting patterns at the nuclear level. The analysis allowed the team to extract predictions for the numbers of protons and neutrons in correlated pairs for different nuclei.
Heavier nuclei, such as gold and lead, contain many more neutrons than protons. Despite this, the team found that the protons and neutrons dominantly form proton-neutron short range correlated pairs, not neutron-neutron or proton-proton pairs. This pattern had already been shown in nuclear physics data, so seeing it replicated here in a model built on the quark-gluon description of nuclei directly links these two realms.
“Even though the number of protons and neutrons were different, if you calculate the numbers that seem to be participating in this pairing, that comes out the same,” Michael Klasen said. “The experimental results are trying to tell us something. What it is, we don’t know yet.”
The result also offers a method for further verifying this model of nuclear structure with future experiments at Jefferson Lab’s Continuous Electron Beam Accelerator Facility. CEBAF is a particle accelerator and DOE Office of Science user facility for nuclear physicists studying the details of nuclear matter. Many details of short-range correlations and their behavior in nuclei have been measured and confirmed with CEBAF.
Now, this model offers the opportunity to make predictions for short-range correlations in nuclei that have so far not been measured but could be verified in future CEBAF experiments.
Further, the model offers the possibility of revealing more about the details of nuclear structure.
As mentioned earlier, the makeup of different atomic nuclei can vary wildly. Some are made up of equal amounts of protons and neutrons, while other nuclei exhibit large disparities between the two types. Certain nuclei have a “magic number” of protons or neutrons, which form a complete shell and increase the stability of these nuclei.
Previous approaches were more generalized and didn’t account for magic numbers and other special characteristics of nuclei. Instead of generalizing, the new framework takes these properties into account, which will allow physicists to consider how the distinct properties of individual nuclei affect the distributions of their constituent quarks and gluons.
“I think the biggest advancement of this work is how we are able to model nuclei in these types of calculations,” Olness said.
The framework could be used for different types of experiments that need to model nuclei. Data from these experiments will also help increase the precision of the model, which has limited measurements on certain nuclei, and test the extracted distributions of short-range correlated pairs.
“This insight has wide-ranging implications, particularly for high-energy heavy-ion and neutrino physics, and will significantly impact experiments at the upcoming Electron-Ion Collider,” Hen said. Hen is also the current chair of the 1,400 members-strong Electron-Ion Collider users group.
The Electron-Ion Collider (EIC), a joint project between Jefferson Lab and DOE’s Brookhaven National Laboratory, is a particle accelerator that will be built at Brookhaven Lab in Long Island, New York. The EIC will collide electrons with atomic nuclei to better our understanding of the building blocks of matter.
Interpreting the EIC’s high-precision nuclear measurements will require high-precision equations of nuclear structure, and this framework is a promising candidate in that quest.
Further Reading
Study shows influence of elementary particles on the structure of atomic nuclei
Correlated Nucleons may Solve 35-Year-Old Mystery
Particles Pick Pair Partners Differently in Small Nuclei
Researchers Overcome the Space Between Protons and Neutrons to Study Heart of Matter
Protons Hog the Momentum in Neutron-Rich Nuclei
Protons Pair Up with Neutrons
-end-
Jefferson Science Associates, LLC, manages and operates the Thomas Jefferson National Accelerator Facility, or Jefferson Lab, for the U.S. Department of Energy’s Office of Science. JSA is a wholly owned subsidiary of the Southeastern Universities Research Association, Inc. (SURA).
DOE’s Office of Science is the single largest supporter of basic research in the physical sciences in the United States and is working to address some of the most pressing challenges of our time. For more information, visit https://energy.gov/science
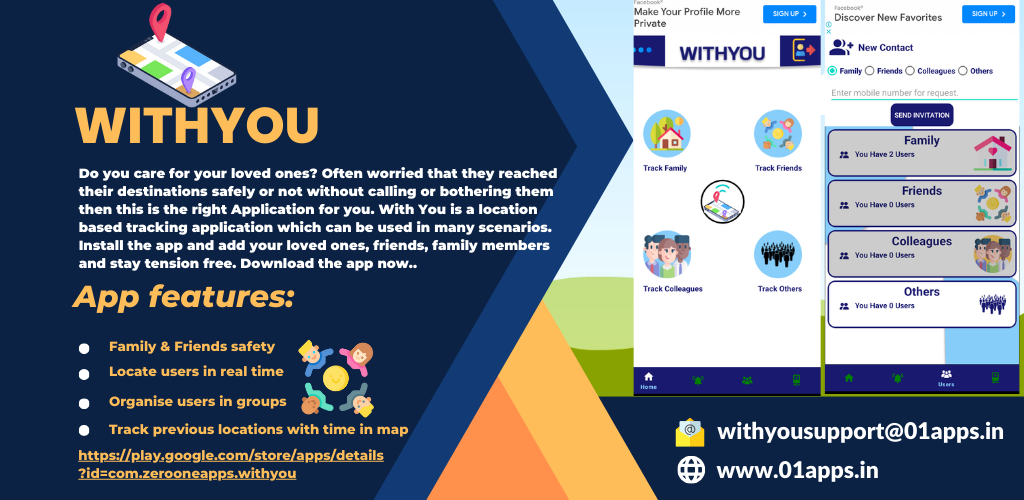