Led by Izabela Szlufarska, a professor of materials science and engineering at UW–Madison, the researchers published details of their work on June 29 in the journal Nature Materials.
Using a combination of experimental characterization and simulations, the team identified potential strategies for encouraging shear bands. This could lead to new ways of increasing the toughness of a wide array of materials.
“In a previous paper, we demonstrated that shear bands in a material called samarium cobalt could actually be beneficial,” says Szlufarska. “That led to the questions, ‘When do shear bands form?’ and ‘When do they support plasticity versus fracture? When do you want to avoid them and when do you want to promote them?’”
In materials with crystalline structures, like metals and ceramics, plasticity is determined by small structural irregularities within the crystal lattice called dislocations. These dislocations can move through the lattice and provide some give, which allows materials like metals to bend without ripping apart the bonds in their rigid structure. However, the more these dislocations are locked into place, either through hardening techniques or natural structural variations, the more brittle a material becomes.
That’s why Szlufarska and her team were surprised to find that in samarium cobalt, a brittle intermetallic material used to make strong magnets, amorphous, or unstructured, shear bands increased the material’s plasticity and were not a sign of failure. Instead, these areas acted as a lubricant, allowing planes of atoms to slide over one another without creating a fracture.
The team hypothesized that these types of beneficial shear bands can form in materials that easily transition between crystalline and amorphous phases. To test this, they looked at aluminum samarium, a glassy material studied extensively by Szlufarska and her colleagues in the NSF-supported Materials Research Science and Education Center at UW–Madison. Using atomic-level simulations, Szlufarska’s group predicted that the crystalline form of this material should also form shear bands under stress. They not only confirmed the finding in the lab, but also varied the atomic composition of the aluminum samarium, making versions where shear bands led either to fracture or to plasticity.
That understanding led the team to propose criteria for screening new materials that might exhibit similar properties and for identifying when shear bands are beneficial. The hope is that these parameters will make it possible to search databases to identify materials that could benefit from doping or engineering to promote shear band formation.
“Increasing toughness, or the amount of energy or stress a material can take before it breaks, by two or three or four times could be really powerful,” says Szlufarska. “In this paper, we extended our finding to a new class of materials. But this is not the end.”
The team intends to test traditional structural materials like oxides, carbides and borides to determine how they can be optimized.
“We understand so much more about how this happens and what to look for,” says Szlufarska. “I think we have identified the key to the design of these materials, and we want to now take them to other classes.”
This work was supported by Army Research Office Grant # W911NF2110130.
Izabela Slurfarska is the Harvey D. Spangler Professor of Engineering and chair of the Department of Materials Science and Engineering. Other UW–Madison authors include Xuanxin Hu, Nuohao Liu, Vrishank Jambur, Siamak Attarian, Ranran Su, Hongliang Zhang, Jianqi Xi and Professor John Perepezko. Other authors include Hubin Luo of the Chinese Academy of Sciences.
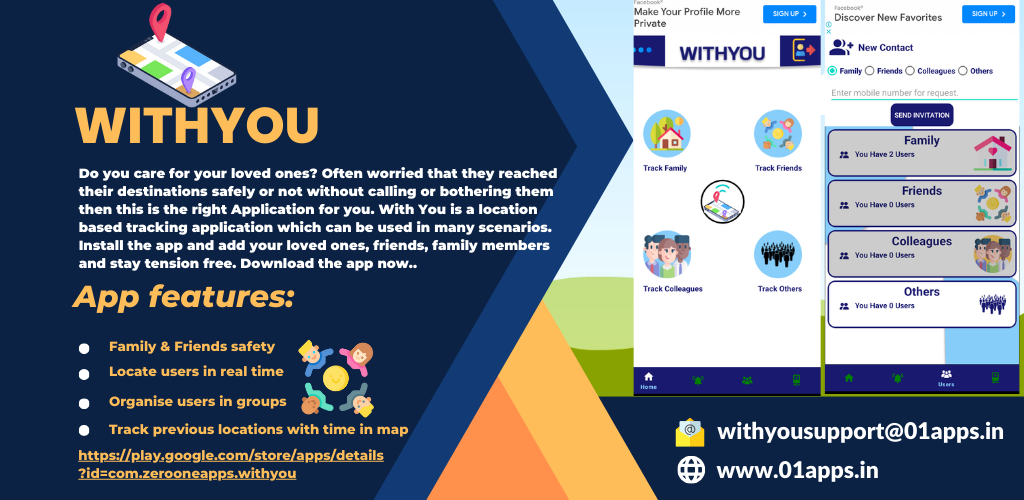