In addition to uncovering a new strategy used by bacteria to protect themselves against viral infection – a strategy that is surprisingly similar to that employed by the immune systems of plants – the research revealed many new retrons that may, in the future, add to the genome-editing toolkit.
The study, conducted in the lab of Prof. Rotem Sorek of the Institute’s Department of Molecular Genetics, was led by lab members Adi Millman, Dr. Aude Bernheim, and Avigail Stokar-Avihail. Prof. Sorek and his team did not set out to solve the retron mystery; rather, they were seeking new elements of the bacterial immune system – specifically, elements that help bacteria fend off viral infection. The scientists’ search was made easier by their recent finding that genes in bacterial immune systems tend to cluster together in the genome within so-called “defense islands.” When they uncovered the unique signature of a retron within a bacterial defense island, the team decided to investigate further.
Their initial research showed that this retron was definitely involved in protecting bacteria against the viruses known as phages that specialize in infecting bacteria. As the researchers looked more closely at additional retrons located near known defense genes, they found that the retrons were always connected – physically and functionally – to one other gene. When either the accompanying gene or the retron was mutated, the bacteria were less successful in fighting off phage infection.
The researchers then began looking for more such retron-gene complexes in defense islands, eventually identifying some 5,000 retrons, many of them new, in different defense islands of numerous bacterial species.
To check whether these retrons function, generally, as immune mechanisms, the researchers transplanted many retrons, one by one, into retron-free laboratory bacterial cells. As suspected, in a great number of these cells they found retrons protecting the bacteria from phage infection.
How do retrons do this? Focusing on one particular type of retron and tracing its actions in the face of phage infection, the researchers discovered that its function is to cause the infected cell to commit suicide. Cell suicide, once thought to belong solely to multicellular organisms, is a last-ditch means of stopping widespread infection – if, that is, the suicide mechanism works fast enough to kill the cell before the virus finishes making copies of itself and spreading to other cells.
Further investigation showed that retrons do not sense the phage invasion itself but, rather, keep watch on another part of the immune system known as RecBCD, which is one of the bacterium’s first lines of defense. If it realizes that the phage has tampered with the cell’s RecBCD, the retron activates its program through the second, linked genes to kill the infected cell and protect the rest of the colony.
“It’s a clever strategy, and we found it works in a similar way to a guard mechanism employed in plant cells,” says Prof. Sorek. “Just like viruses that infect plants, phages come equipped with a variety of inhibitors to block assorted parts of the cell immune response. The retron, like a guard mechanism known to exist in plants, does not need to be able to identify all possible inhibitors, just to have a handle on the functioning of one particular immune complex. Infected plant cells apply this ‘local suicide’ method, killing off a small region of a leaf or root, in an effort to save the plant itself. Since most bacteria live in colonies, this same strategy can promote the survival of the group, even at the expense of individual members.”
Retrons are very useful to biotechnology because they begin with a piece of RNA, which is the template for the synthesis of the DNA strand. In the retron sequence, this template can be swapped out for any desired DNA sequence and used – sometimes in conjunction with CRISPR, another tool borrowed from the bacterial immune toolkit – to manipulate genes in various ways. Prof. Sorek and his team believe that the diverse list of retrons they identified may contain more than a few able to provide better templates for specific gene-editing needs.
Prof. Rotem Sorek’s research is supported by the Knell Family Center for Microbiology, which he heads; the Willner Family Leadership Institute for the Weizmann Institute of Science; the Sagol Weizmann-MIT Bridge Program; the Schwartz/Reisman Collaborative Science Program; the Ben B. and Joyce E. Eisenberg Foundation; the Yotam Project; and the European Research Council.
The Weizmann Institute of Science in Rehovot, Israel, is one of the world’s top-ranking multidisciplinary research institutions. The Institute’s 3,800-strong scientific community engages in research addressing crucial problems in medicine and health, energy, technology, agriculture, and the environment. Outstanding young scientists from around the world pursue advanced degrees at the Weizmann Institute’s Feinberg Graduate School. The discoveries and theories of Weizmann Institute scientists have had a major impact on the wider scientific community, as well as on the quality of life of millions of people worldwide.
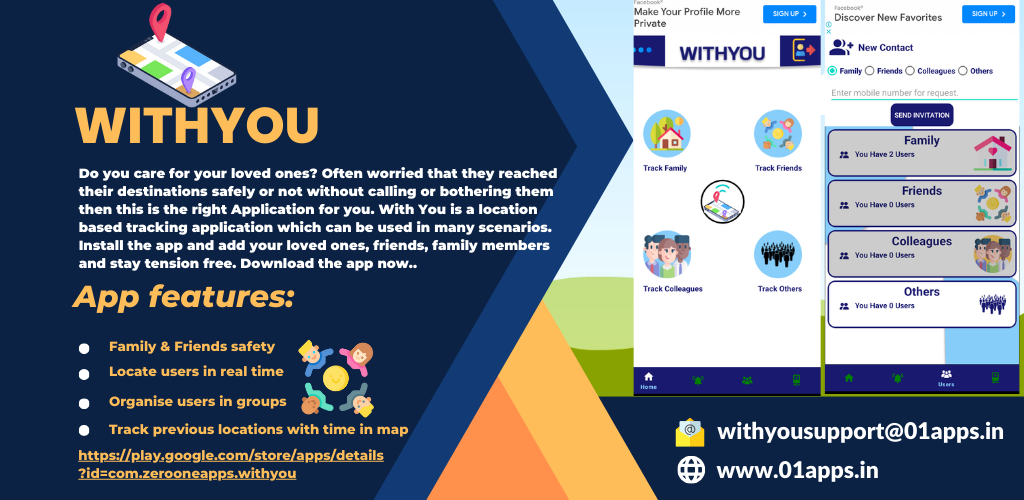