Deep Forest Soils Lose Carbon as Temperatures Climb
Study shows even the large, old, and presumably stable stores of soil carbon are vulnerable to warming and could form positive feedbacks to climate change
-By Julie Chao
A new study led by Berkeley Lab has provided the first physical evidence that warmer temperatures lead to a significant drop in the stored carbon stock in deep forest soils. An experiment in California’s Sierra Nevada forest found that the carbon content in subsoils dropped 33% over five years.
The research team, led by Margaret Torn, artificially heated plots of soil down to 1 meter deep by 4 degrees Celsius, which is the amount of heating projected by century’s end in a business-as-usual climate scenario. (The heating followed natural diurnal and seasonal cycles.) “We heated the whole soil profile, whereas most experiments just heat the surface,” Torn said. “That deeper soil has a very large stock of carbon and because it’s old people have assumed that it’s also stable. But we’re saying, nope, actually, you can lose this deep, older carbon.”
This study was published in Science Advances, with Jennifer Soong as the lead author.
Soils contain twice as much carbon as the atmosphere does, and deeper subsoils (more than 20 centimeters) are believed to account for roughly half of the soil carbon. The same research team found in 2017 that the warmed soil led to an increase of CO2 released into the atmosphere by 34 to 37%.
The new study confirms that this release has continued unabated five years later and provides physical evidence of the net transfer of carbon from the soil, where it was sequestered as organic carbon, to the atmosphere, released as a greenhouse gas. This cycle, if confirmed over longer time scales, could constitute a positive feedback to climate change.
“Forests are currently a net sink of carbon and still have the potential to sequester large amounts of atmospheric CO2 in coming decades,” Torn said. “However, a better understanding of how deep soils will respond to warming is needed to accurately predict and plan for long-term changes.”
Emissions from Vaporizable Cannabis Concentrates Have Potential Health Risks
Berkeley Lab researchers analyze vaping emissions from non-cannabinoid substances found in the cannabis plant
-By Kiran Julin
As more U.S. states and countries legalize medical and recreational marijuana, consumers are increasingly turning to new types of products that avoid toxic smoke inhalation. Researchers at Berkeley Lab who previously identified potentially harmful emissions from electronic cigarettes are now identifying the potential health risks of vaping cannabis.
When a person vapes marijuana, they are inhaling vaporizable cannabis concentrate (VCC), which is a concentrated liquid or a waxy solid form of cannabis also commonly referred to as “extracts” or “hash oils.” The concentrated liquid is usually heated into an aerosol by a battery-operated electronic device, while waxy concentrates can be aerosolized by direct application onto a heated surface (known as “dabbing”).
In a new Berkeley Lab study recently published in the journal Environmental Science & Technology, researchers examined emissions associated with heating only the non-cannabinoid constituents of VCC, which are extracted from the cannabis plant alongside the psychoactive constituents but do not cause drug-like effects on the body. These non-cannabinoid compounds are a major part of VCC formulations and include terpenoids, flavonoids, and lignins – all commonly found in plants and potentially harmful when inhaled.
The research team, led by Berkeley Lab scientist Hugo Destaillats, heated and aerosolized mixtures of those compounds to simulate cannabis vaping and dabbing. They observed the formation of a large number of ultrafine particles that were released into a room-sized chamber and remained airborne for at least three hours.
“Our results suggest that high molecular weight compounds such as lignins, flavonoids, and triterpenes enhance the formation and accumulation of ultrafine particles in the air, which can then serve as carriers of substances that normally are not found in the air – otherwise known as nonvolatile and semivolatile species,” said Berkeley Lab scientist and co-author Xiaochen Tang. “These substances can then be breathed into a person’s lungs and found in the indoor environment.”
The research team also quantified 11 degradation byproducts as a result of heating the mixtures, including acrolein and methacrolein, which are highly irritating to eyes and the respiratory system. According to the study, these compounds are predicted to reach levels that may exceed reference exposure levels recommended by California.
“The bottom line is that emissions from heated cannabis concentrates are not innocuous,” said Destaillats. “Vaping and dabbing can be a source of harmful chemical exposure, and more research is needed to determine the full extent of the risk.”
The study was funded by the University of California Tobacco Related Diseases Research Program (UC-TRDRP).
Scientists Analyze Dry-sanitizing Device for Reusable PPE
The new device appears promising for decontaminating multiple types of personal protective equipment
-By Ashleigh Papp
Ending the COVID-19 pandemic will require many things — widespread vaccine rollout and treatment options, as well as a stable supply of personal protective equipment (PPE) like N95 face masks and plastic face shields. Today, most PPE is used only once, which has created an unsustainable strain on production lines and a massive spike in landfill loads.
Current methods to decontaminate PPE, like ultraviolet germicidal irradiation, heat sterilization, and chemical disinfectants, are successful at eradicating the virus but compromise the PPE material, ultimately increasing the wearer’s potential exposure to pathogens.
Antoine Snijders, a staff scientist at Berkeley Lab, and collaborators at the University of Nevada, Reno (UNR) School of Medicine have confirmed that under the conditions tested, a new dry-sanitizing device may provide a safe and reliable solution that doesn’t alter the integrity of the PPE material. They reported their results in the journal Pathogens.
Snijders worked with his UNR colleagues to design the experiments that evaluated the efficacy of this device on N95 face masks (made of a porous material) and face shields (made from nonporous material). The device, about the size of a medium-sized trash bin, uses air pressure and ionization to decontaminate viruses and bacteria. Both forms of PPE were exposed to a close genetic model of the SARS-CoV-2 virus and put inside the device for 15 minutes. Subsequent tests indicated that the virus had been successfully inactivated on both materials.
Snijders’s contribution to the research was supported by the Department of Energy’s Office of Technology Transitions.
Read the UNR press release.
New Tools Link Catalytic Activity to Nanoscale Transformations
Scientists discovered exactly how a catalyst splits water molecules, an important step in improving the efficiency of hydrogen fuel generation
-Adapted from SLAC news release by Glennda Chui
Transitioning to a clean hydrogen economy will require cheaper, more efficient ways to use renewable sources of electricity to break water into hydrogen and oxygen. But a key step in that process, known as the oxygen evolution reaction, has proven to be a bottleneck. Today it’s only about 75% efficient, and the catalysts used to accelerate the reaction, like platinum and iridium, are rare and expensive.
To address these issues, an international team led by scientists at Stanford University and SLAC National Accelerator Laboratory developed a suite of advanced tools, including a new apparatus at Berkeley Lab’s Advanced Light Source (ALS) that enables X-ray microscopy of catalysts under working conditions. The results were published recently in Nature.
The full suite of techniques, which also included electrochemical atomic force microscopy and scanning electrochemical cell microscopy, allowed the researchers to zoom in on tiny, six-sided nanoparticles of the catalyst, cobalt oxyhydroxide, and watch them accelerate the generation of oxygen.
At the ALS, an electrochemical flow cell was integrated into an X-ray beamline used for scanning transmission X-ray microscopy (STXM). This allowed the researchers to map out the oxidation state of the working catalyst – a chemical state associated with catalytic activity – in areas as small as about 50 nanometers (50 billionths of a meter) in diameter.
The researchers discovered that most of the catalytic activity occurred at the particle edges, and they were able to observe the chemical interactions between the particle and the surrounding liquid at the nanometer scale as they turned up the voltage to drive the reaction. Combining their observations with prior computational work, they were able to identify a single step in the reaction that limits how fast it can proceed.
“This suite of methods can tell us the ‘where, what, and why’ of how these electrocatalytic materials work under realistic operating conditions,” said Tyler Mefford, a staff scientist with Stanford and the Stanford Institute for Materials and Energy Sciences (SIMES) at SLAC who led the research. “Now that we have outlined how to use this platform, the applications are extremely broad.”
More:
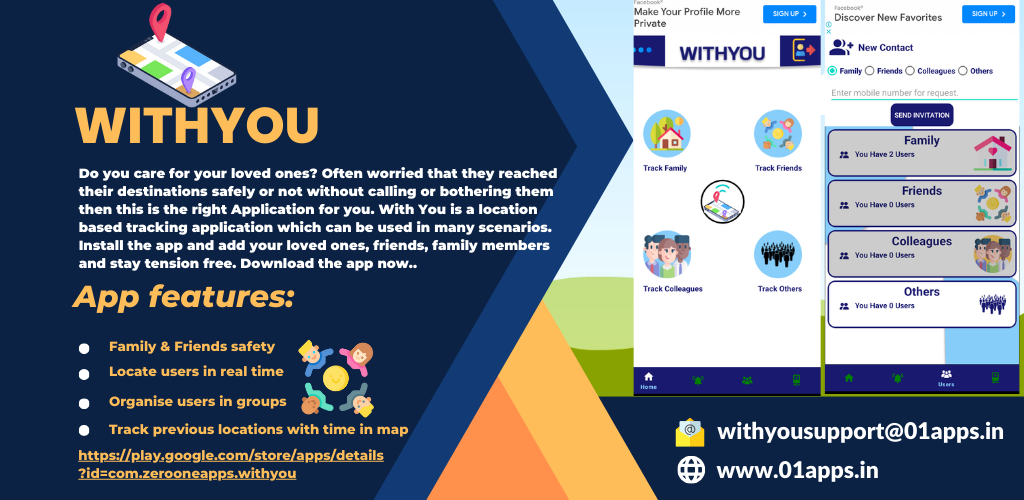