Now, University of Maryland School of Medicine and University of Vermont researchers have shown how the brain communicates to blood vessels when in need of energy, and how these blood vessels respond by relaxing or constricting to direct blood flow to specific brain regions.
In their new paper, published on July 21 in Science Advances, the researchers say that understanding how the brain directs energy to itself in intricate detail can help determine what goes wrong in conditions like Alzheimer’s disease and dementia, where faulty blood flow is a predictor for cognitive impairment. If the brain does not get blood to where it needs it when it needs it, the neurons become stressed, and over time they deteriorate ultimately leading to cognitive decline and memory problems.
Large arteries feed medium-sized vessels known as arterioles that then feed even tinier capillaries—so small that only a single blood cell can pass through at once. In a 2017 Nature Neuroscience paper, the researchers showed that electrical pulses coursing through the capillaries direct blood flow from the medium-sized arterioles supplying large regions of the brain. For this latest paper, the team wanted to study the fine-tuning of blood as it flows through the capillaries to precisely regulate energy supply to tiny regions in the brain.
“There seem to be two mechanisms working in tandem to ensure that energy in the form of blood makes it to specific regions of the brain: one broad and the other precise,” says Thomas Longden, Ph.D., Assistant Professor of Physiology at University of Maryland School of Medicine. “The first electrical mechanism is like a sledgehammer approach to get more blood to the general vicinity of the increased brain activity by controlling the medium-sized arterioles, and then capillary calcium signals ensure exquisite fine-tuning to make sure the blood gets to exactly the right place at the right time through the tiny capillaries.”
Dr. Longden and his collaborators used a protein which emits green light when calcium increases in the cell. Due to the efforts of Michael Kotlikoff’s team at Cornell University, they were able to turn this tool on in the cells lining blood vessels of mice. The researchers then looked through little windows in the brains of these mice to investigate calcium’s role in controlling blood flow in the brain’s capillaries. When the cells lining the blood vessels received an influx of calcium, they glowed green. They detected 5,000 calcium signals per second in the capillaries in the tiny section of brain visible through the window, which they say amounts to about 1,000,000 of these responses each second in the entire brain’s blood vessel system.
“Until we deployed this new technology, there was this wholly unseen world of calcium signaling in the brain hidden from view, and now we can see a ton of activity within the brains blood vessels – they are constantly firing,” says Dr. Longden.
Dr. Longden and the research team then dissected the intricate cellular mechanism behind calcium’s role in directing blood branch-by-branch through the tiny vessels of the brain. They found that when neurons fire electrical signals, they cause an increase in calcium in the cells lining the blood vessels. Then enzymes detect this calcium and direct the cells to make nitric oxide. Nitric oxide is a hormone (and a gas) that causes muscle-like cells around blood vessels to relax, which then widens the vessels allowing more blood to flow in.
“Capillaries were traditionally thought as simple conduits for red blood cells, and the barrier between the blood and brain,” says co-senior author Mark T. Nelson, PhD, University of Vermont Distinguished Professor and Chair of Pharmacology. “Here, we revealed an unknown universe of calcium signaling in capillaries, and much like traffic lights, these calcium signals direct vital nutrients to nearby active neurons.”
“The first step towards figuring out what goes wrong in diseases is to determine how the system works as it normally should,” says E. Albert Reece, MD, PhD, MBA, Executive Vice President for Medical Affairs, UM Baltimore, and the John Z. and Akiko K. Bowers Distinguished Professor and Dean, University of Maryland School of Medicine. “Now that the researchers have a handle on how this process works, they can begin to investigate how the blood flow is disrupted in Alzheimer’s disease and dementia in order to figure out ways to fix it.”
This study was supported by the American Heart Association, the Totman Medical Research Trust, Fondation Leducq, European Union’s Horizon 2020 research and innovation programme, the Henry M. Jackson Foundation for the Advancement of Military Medicine, the National Institute of Heart, Lung, and Blood (P01-HL-095488, R01-HL-121706, R37-DK-053832, 7UM-HL-1207704, R01-HL-131181, R24HL120847, R01HL120323, and R35HL140027), the National Institute of General Medical Sciences (4P20 GM103644/4-5, P20-GM-135007), the National Institute of Neurological Disorders and Stroke (R01NS110656), the National Institute on Aging (R01AG066645), the Vermont Center for Cardiovascular and Brain Health, the University of California Irvine TMF Center supported by the National Cancer Institute (P30-CA062203), and the German Research foundation (DFG)-funded Research Unit.
Dr. Longden is a recent recipient of the NIH Director’s New Innovator Award from the National Institutes of Health Office of the Director (DP2OD02944801).
More information about the work ongoing in his lab can be found at longdenlab.org.
The authors have no financial conflicts to report.
About the University of Maryland School of Medicine
Now in its third century, the University of Maryland School of Medicine was chartered in 1807 as the first public medical school in the United States. It continues today as one of the fastest growing, top-tier biomedical research enterprises in the world — with 45 academic departments, centers, institutes, and programs; and a faculty of more than 3,000 physicians, scientists, and allied health professionals, including members of the National Academy of Medicine and the National Academy of Sciences, and a distinguished two-time winner of the Albert E. Lasker Award in Medical Research. With an operating budget of more than $1.2 billion, the School of Medicine works closely in partnership with the University of Maryland Medical Center and Medical System to provide research-intensive, academic and clinically based care for nearly 2 million patients each year. The School of Medicine has more than $563 million in extramural funding, with most of its academic departments highly ranked among all medical schools in the nation in research funding. As one of the seven professional schools that make up the University of Maryland, Baltimore campus, the School of Medicine has a total population of nearly 9,000 faculty and staff, including 2,500 student trainees, residents, and fellows. The combined School of Medicine and Medical System (“University of Maryland Medicine”) has an annual budget of nearly $6 billion and an economic impact more than $15 billion on the state and local community. The School of Medicine, which ranks as the 8th highest among public medical schools in research productivity, is an innovator in translational medicine, with 600 active patents and 24 start-up companies. The School of Medicine works locally, nationally, and globally, with research and treatment facilities in 36 countries around the world. Visit medschool.umaryland.edu
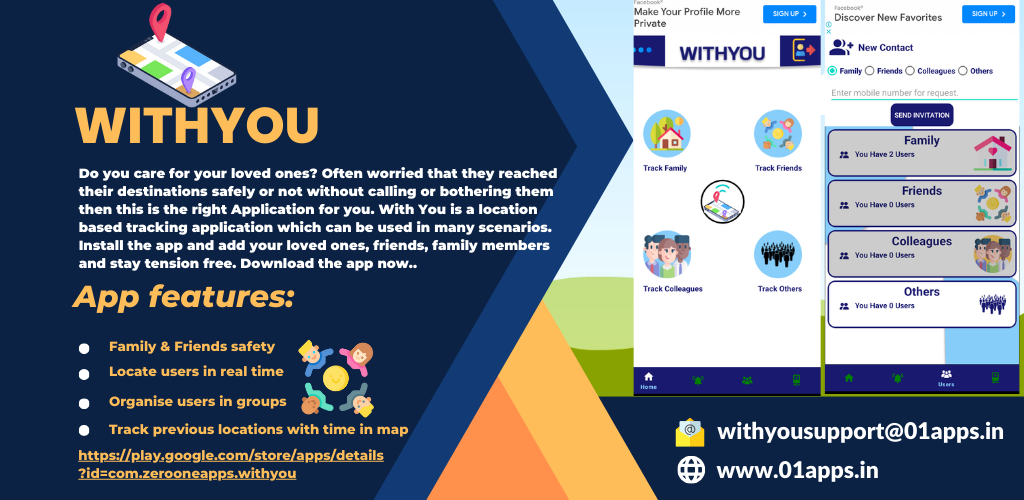