In the First-Person Science series, scientists describe how they made significant discoveries over years of research. Christoph Benning is the director of the Michigan State University-Department of Energy Plant Research Laboratory.
“We have to show Mr. Benning how to purify chloroplasts. I think the purification wasn’t quite right,” mocked a fellow senior researcher as I stood on the podium. I had just finished a presentation at the International Symposium on Plant Lipids, the major conference in my research area. I had described my laboratory’s finding that a certain type of lipid – a biological molecule that includes fatty acids and sugar – had built up in plant roots. No one had ever found this lipid in roots before. So he was skeptical.
He was also wrong. We published the paper in the Proceedings of the National Academy of Sciences. Later on, other scientists confirmed our findings.
It was just one of the surprising discoveries we made in researching these lipids, all funded by the Department of Energy’s (DOE) Office of Science. Unlike the typical story of “develop hypothesis,” “test hypothesis,” “get results,” this story had many twists and turns. Questions that we thought of early on weren’t answered until years later, often serendipitously. Nonetheless, this is some of the best work I’ve ever done, first as a faculty member at Michigan State University (MSU) and then as the director of the MSU-DOE Plant Research Laboratory.
The Powerhouses of Plants
Our work examines the materials in the membranes of chloroplasts, the compartments plant cells use for photosynthesis. These membranes are essential for life on Earth.
The proteins and pigments that convert light to chemical energy inside these membranes are embedded in lipids. Our research looked at a group of lipids called galactolipids, which make up about 70 percent of the membranes in green plants. When we remove these lipids, plants become small and sick.
Even though these lipids are the most abundant in the biosphere, they don’t get a lot of attention in the scientific community. Because they’re not in animal cells, textbooks don’t mention them much. But I find their biochemistry really interesting.
To understand how plants make these lipids, we studied a plant called Arabidopsis. Related to cabbage and mustard greens, it’s considered the “standard reference” for all plants.
Strange Mutants: The Mid-1990s
When I arrived at Michigan State University in 1998, I focused on a mutant version of Arabidopsis called dgd1. Because of a mutation in one of its genes, it’s missing 90 percent of a specific galactolipid in its chloroplast membranes. We call this galactolipid, DGDG. The mutant plant is tiny; it’s obvious the mutation has a big effect on growth. But we didn’t know which gene was broken or what processes that gene affected.
These days, it’s not too hard to link a physical characteristic in a mutant to the gene defect responsible for that characteristic. Scientists deciphered Arabidopsis’ entire genome in 2000. But in 1998, we didn’t have that information. Instead, we mapped the mutation to find the broken gene, which took three years. It was a huge effort to find that one single mutation.
Initially, we proposed that the enzyme made DGDG by moving sugar molecules between lipids. But later on, my co-author, Peter Dörmann, figured out that this particular enzyme didn’t have that function. So what was moving those sugars between lipids? That remained a mystery until much later on.
Denying an Important Element: 2000
When scientists mapped the full Arabidopsis genome in 2000, we realized there was a second gene involved in making the DGDG galactolipid: DGD2. But we didn’t know what it did.
The answer started to show itself when we looked at what happens when you deny phosphorus to Arabidopsis. Phosphorus is very important for plants; farmers use phosphorus in fertilizers to boost growth. But unlike many other lipids, the plant doesn’t need phosphorus to produce DGDG. From previous research, we knew that when plants can’t access phosphorus, they change the types of lipids in their chloroplast membranes.
One of my postdocs, Heiko Härtel, clever as he was, denied phosphate to a dgd1 mutant of Arabidopsis that couldn’t make DGDG in its chloroplasts. But despite the original dgd1 mutation, the phosphate-starved plant produced quite a bit of DGDG! He realized “Whoa – this mutant gene’s effects can be suppressed!” Whereas the dgd1 mutant only had 10 percent of the DGDG levels compared to normal, the phosphate-starved dgd1 mutant had 60 percent. Responding to the lack of phosphorus, the mutant plant changed the pathway it used to make the lipid. My former co-worker Peter Dörmann later found that the DGD2 gene was responsible for this alternative pathway.
But that wasn’t the only surprise. Looking at the chemicals in the phosphate-starved plant’s roots, we found a bunch of DGDG. And that couldn’t be. Roots typically don’t have chloroplasts. That prompted us to think, “Hmmm, wait a minute. Maybe this stuff isn’t just in the chloroplasts. Maybe the plant has moved it under these conditions.”
That’s actually what happens. When we denied regular Arabidopsis phosphate, more and more of this lipid showed up, especially in the roots.
We realized that when the plant doesn’t have enough phosphate to make phosphate-based lipids, it starts replacing them with lipids that don’t need phosphate, like DGDG. If plants relied on having access to phosphate to build these essential membranes, they’d face big problems when there’s a lack of it. The ability to substitute lipids ensures plants have the material they need to make these membranes.
This was the result that led to the researcher calling me out at the conference in 2000. The lesson I learned: When you run into dogmatic people, don’t be intimidated. Just do your own thing. This is how science can advance.
Since then, other scientists have found proof in nature of the relationship between phosphorus and non-phosphorous lipids. A scientist with Woods Hole Oceanographic Institution, Bejamin Van Mooy, contacted me. He had taken samples on a ship crossing the Pacific Ocean from north to south and said, “I came across your papers and this is real.” There’s very little phosphorus in the ocean, so these non-phosphorous lipids are very important for marine plankton.
Accidently Finding a Lipid Transporter: 2003
Knowing that Arabidopsis had two different ways of making DGDG, we wanted to figure out exactly how phosphorus influenced that dynamic. To pinpoint how the plant was switching between the two different ways, we searched for a plant with two mutations. The first was the dgd1 mutation that led to the plant not producing DGDG in the chloroplasts; the second mutation would remove the switch, or so we thought.
Instead, we ended up finding an unrelated machinery that was indirectly leading the dgd1 mutant plant to accumulate DGDG. I said to my postdoc, Changcheng Xu, “I think it isn’t moving lipids from one place to another,” referring to the new mutant he found. He responded, “No way, no way.” Despite his skepticism, he searched for the gene and the protein it produces. And he found it – the first TDG protein.
It turned out that this protein was part of a group of proteins that move lipids into chloroplasts that researchers had been trying to find for decades. While they recognized the process, they didn’t know the proteins involved. We just happened upon one of them! Xu went on to DOE’s Brookhaven National Laboratory where he identified another protein in the same group. Even though we were looking for the phosphate regulation switch, we ended up discovering a big, other thing.
When we then disabled the genes that produced the machinery that was importing lipids into the chloroplasts, the plant activated a different enzyme that also helped produce lipids. This one we called PGT. As it turned out, this was the enzyme we were looking for in the first experiment almost 10 years earlier! This new PGT enzyme moved the sugar galactose from one galactolipid to another. In the process, it made DGDG and other galactolipids. But there was still more for us to learn about it.
Not So Sensitive to Freezing: 2010
One day, I was reading a journal article that described a mutation that makes plants sensitive to freezing.
When plant cells freeze, water forms ice crystals. The crystals pull water out of the chloroplasts, which dehydrates and shrinks them. When the membranes shrink, they can’t squish like the inner tube of a soccer ball. Instead, they fuse and get damaged. To prevent this damage, plants can remove lipids and store them away in a more stable form. You can see these vegetable oil droplets accumulating on the chloroplasts’ outer membranes.
After reading the paper, I made a casual comment to one of my students, Eric Moellering. I asked him, “Why don’t you check out whether this mutation is in the gene we’re looking for?” I had a hunch that the protein missing in the freezing-sensitive mutant looked right — like something we had seen before. The gene I was referring to was the one that codes for the PGT enzyme that moved around sugars that we had seen earlier.
Then I forgot about it.
Until Moellering came back in my office yelling, “We found it!” I said, “What do you mean?” He said, “You found it! This is the PGT.” The enzyme we had looked for all of those years ago – the one that that moves the sugar galactose from lipid to lipid – is also the enzyme that helps plants survive freezing. The process of moving the galactose allows the plant to make more stable lipids.
Finding the gene and enzyme that protects plants from freezing took 10 years of work. Researchers had started investigating this problem more than 30 years ago, but they didn’t have the technology to find the gene. Our first paper had most of the process right, except we incorrectly described the activity of the enzyme. But that’s science. You don’t always have it perfect in the first round.
Digging into How Plants Protect Themselves: 2010-Now
We’re still investigating the mechanism driven by the PGT enzyme that protects plants from freezing. Climate change is causing warmer temperatures earlier in spring, which causes trees to flower. But they can still get hit by a strong, unexpected freeze. So it would be very useful to have mechanisms that could protect plants against short-term freezing.
The PGT enzyme plays a big role in protecting against other forms of stress as well. The same process protects plants from drought or being exposed to salt. Besides agriculture, these findings can help us more effectively grow plants for biofuels.
All of these discoveries came about because my team and I were open to going down unusual research paths following our intuition and had the support to do so. I’ve learned over the course of my career not to pooh-pooh anyone who comes in with strange-looking data. Ninety-nine percent of the time, they’ve discovered something novel and they haven’t realized it. I can point out to them: “You’ve discovered something really big here!” That collaborative work and resulting discoveries are what bring me the most satisfaction in my job.
The Office of Science is the single largest supporter of basic research in the physical sciences in the United States and is working to address some of the most pressing challenges of our time. For more information please visit https://www.energy.gov/science.
Original post https://alertarticles.info
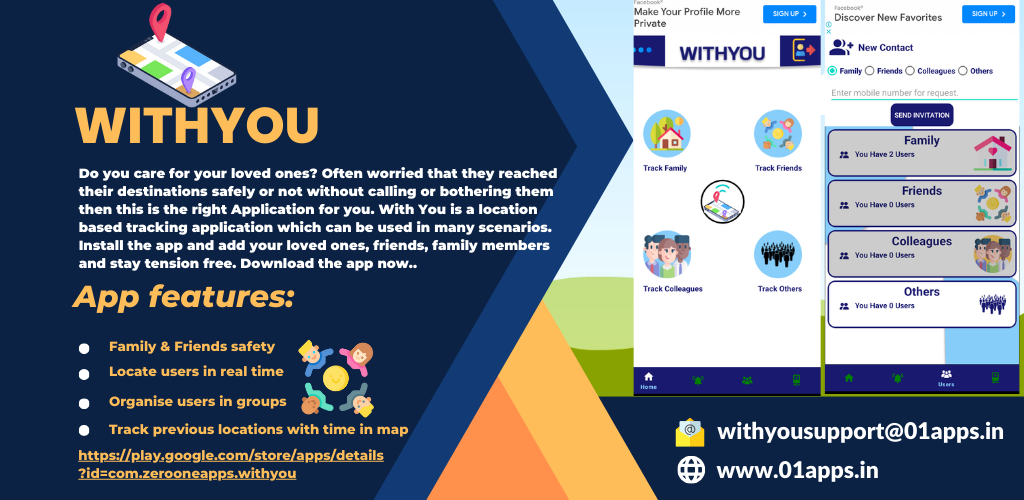