We experience turbulence every day: a gust of wind, water gushing down a river or mid-flight bumps on an airplane.
Although it may be easy to understand what causes some kinds of turbulence — a felled tree in a river or a bear splashing around for salmon — there is now evidence that a very small disturbance at the start can have dramatic effects later. Instead of a tree, think of a twig — or even the swerving motion of a molecule.
University of California San Diego Chancellor’s Distinguished Professor of Physics Nigel Goldenfeld, along with his former student Dmytro Bandak, and Professors Alexei Mailybaev and Gregory Eyink, has shown in theoretical models of turbulence that even molecular motions can create large-scale patterns of randomness over a defined period of time. Their work appears in Physical Review Letters.
The Butterfly Effect
A butterfly flaps its wings in Brazil which later causes a tornado in Texas. Although we may commonly use the phrase to denote the seeming interconnectedness of our own lives, the term “butterfly effect” is sometimes associated with chaos theory. Goldenfeld said their work represents a more extreme version of the butterfly effect, first described by mathematician and meteorologist Edward Lorenz in 1969.
“What we’ve learned is that in turbulent systems, a very small disturbance at one point will have an amplified effect at a finite point in the future, but through a mechanism that is faster than chaos.”
Although the mathematical mechanism for this amplification, known as spontaneous stochasticity, was discovered about 25 years ago, Goldenfeld noted, “The fact that the random motion of molecules, responsible for the everyday phenomenon of temperature, could generate spontaneous stochasticity was not known before our work.”
Thinking back on the twig in the river: while you might notice a small disturbance where the water flows over the twig, you wouldn’t expect it to create a great deal of turbulence (via eddies and swirls) downstream. Yet that is precisely what Goldenfeld’s paper shows. He explains that the mechanism is known as spontaneous stochasticity, because the randomness arises even though the fluid motion was expected to be predictable. Furthermore, it would be impossible to pinpoint the twig that had originally set the eddies and swirls in motion. In fact, there may be no disturbance in the water flow where the twig is located at all.
The team’s findings also showed that spontaneous stochasticity happens regardless of the initial disturbance. Whether it’s a twig, a pebble or a clod of dirt, the randomness you get on a large scale is the same. In other words, the randomness is intrinsic to the process.
The team used thermal noise as the system for their calculations because it is always present — noticeable in the hissing of your amplifier. That noise is the sound of electrons moving around inside your electronics. In a fluid, it is the molecules that are moving around instead of electrons.
Although the Navier-Stokes equation is the standard model for computing turbulent flows, it was computationally unfeasible to use the full fluid equations to simulate the very extreme turbulent events required to convincingly demonstrate the team’s claims. Instead, they used a simplified equation, and in doing so showed that a disturbance on the scale of microns (a millionth of a meter) could cause entire fluid systems to exhibit spontaneous stochasticity in a way that did not depend on the source of the disturbance.
“For now, this will have to do” said Goldenfeld, “but we hope that future supercomputer calculations will be able to confirm our results using the full fluid equations.”
Predicting the Future and the Past
“There exists a fundamental limit to what can be predicted with turbulence,” stated Goldenfeld. “You see this with weather forecasts; there is always a fundamental source of randomness. The precise sense in which this unpredictability was inevitable wasn’t fully understood before our work.”
It’s that randomness that makes it so hard to accurately predict the weather more than a few hours in advance. Meteorological stations sample weather in select locations and computer simulations stitch them together, but without knowing the exact weather everywhere right now, it’s hard to predict the exact weather everywhere in the future. This paper hints at the possibility that fundamental limits will always exist because randomness will always show up.
There may also be implications in astrophysics research. Scientists already understand that computer simulations of how galaxies are formed and how our universe evolved are sensitive to noise. Often the behaviors of stars, planets and galaxies cannot be easily explained and may be attributed to the kinds of microscopic noise that Goldenfeld and his colleagues have uncovered.
Full list of authors: Dmytro Bandak (University of Illinois at Urbana-Champaign), Alexei Mailybaev (Instituto de Matemática Pura e Aplicada – IMPA, Brazil), Gregory L. Eyink (The Johns Hopkins University) and Nigel Goldenfeld (UC San Diego).
This work was partially supported by the Simons Foundation (663054 and 662985), the National Council for Scientific and Technological Development in Brazil (CNPq grant 308721/2021-7) and the Carlos Chagas Filho Foundation for Research Support in Brazil (FAPERJ grant E-26/201.054/2022).
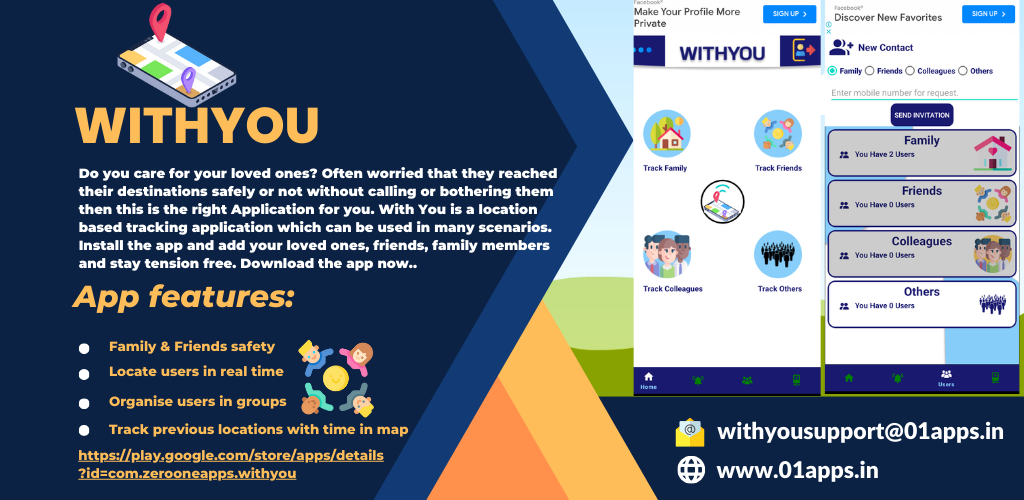