“The atmosphere of our sun consists of a hot gas that is ionized at different levels called plasma,” Yalim notes. “The plasma temperature from the photosphere to corona increases from 5,000 to 1 million degrees Kelvin over a distance of only 10,000 kilometers in the chromosphere. A number of different mechanisms have been introduced over many decades to explain this. Today, it is largely accepted that this heating is strongly related to the magnetic field structure of the sun.”
The chromosphere is a thin layer of plasma between the sun’s visible surface, the photosphere, and the corona, the sun’s upper atmosphere, a region that extends approximately 2,000 km above the surface.
“In this research, together with my project team, I have been investigating a known but not-much investigated mechanism called Joule heating,” Yalim says. “The idea is the same as the traditional Joule heating that we encounter in our daily lives governed by Ohm’s law: an electric current passing through a resistor under a voltage causes heating of the resistor. To be able to talk about this mechanism in terms of the sun, we need electric currents in a plasma environment, and we have strong electric currents in the chromosphere. Together with magnetic resistivity, which is also relatively strong in this layer, Joule heating becomes a significant heating mechanism.”
Gas turns into plasma when heat or energy is added to it, causing the atoms that make up the gas to start to lose their electrons and become positively charged ions. The lost electrons begin to float freely, a process called ionization.
“Temperatures in the chromosphere are not as elevated as in the outer layer of the solar atmosphere, the corona,” Yalim explains. “This leads to a unique environment where the plasma is not fully ionized, leading to the presence of ions, neutral particles and electrons simultaneously, which is called a weakly-ionized plasma. In such a setting, we have a direction-dependent dissipation mechanism for electric currents.”
In physics, “dissipation” refers to how dynamic mechanisms, such as waves or oscillations, lose energy over time, generally due to the action of friction or turbulence. The lost energy is converted into heat, raising the temperature of a system. In heliophysics in particular, dissipation results in two different types of magnetic resistivities: the better-known Coulomb resistivity, which dissipates electric currents that are parallel to magnetic field lines, and Cowling resistivity, the focus of Yalim’s work, where resistivity arises specifically in this type of weakly-ionized plasma found in the chromosphere that dissipates electric currents that are perpendicular to the direction of the magnetic field.
“‘Cowling heating’ is short for Joule heating caused by Cowling resistivity,” the UAH scientist says. “Cowling resistivity enhances the overall resistivity in this solar plasma region significantly. It varies with the amount of neutral particles, temperature, magnetic field strength and density of the plasma, and the combination of all these parameters result in a much higher resistivity, several orders of magnitude larger than the usual Coulomb resistivity found ubiquitously in the solar atmosphere. This results in significant Joule heating rates at levels that can provide the additional heating necessary to obtain the observed temperature distributions.”
In examining this process, Yalim’s team focuses on phenomena within the sun’s chromosphere called “light bridges,” lanes of bright material that often divide the umbra, or central dark region, of a sunspot.
“Just as we need a strong Cowling resistivity to have Cowling heating, we also need a strong perpendicular electric current,” the researcher says. “The conditions in the sunspot umbral light bridges exhibit both of these conditions remarkably well as we showed in our latest paper, making them the primary target for our investigation. Although we focused on a sunspot light bridge in this study, our proposed mechanism can technically occur wherever there are discontinuities in the magnetic field topology that produce electric currents that can heat the plasma in those regions by dissipation through Cowling resistivity.”
Looking to the future, Yalim believes the path forward for this research is clear. “This project investigates this process through an observational study of several NSF and NASA data sets. With the advent of the ground-based and space-borne solar observational capabilities, we can develop data-constrained/data-driven models more confidently to pursue results with unprecedented accuracy. Our next step is to investigate Cowling heating more broadly within the solar chromosphere and possibly in the lower corona in regions where the necessary conditions for this mechanism are favorable.
“These are exciting times to pursue this effort,” Yalim concludes. “The project supports early career scientists, and since 2019 I have served as the program coordinator of our NSF Research Experiences for Undergraduates (REU) efforts in solar and heliospheric physics which is jointly organized by CSPAR and NASA’s Marshall Space Flight Center. I’ve also mentored three undergraduate students from UAH specifically on the topic of this project since 2022. So, this work has also had a significant outreach/educational component. This project is jointly funded by the NSF Solar-Terrestrial Research program and the Established Program to Stimulate Competitive Research (EPSCoR).”
Kristina Hendrix
256-824-6341
[email protected]
Julie Jansen
256-824-6926
[email protected]
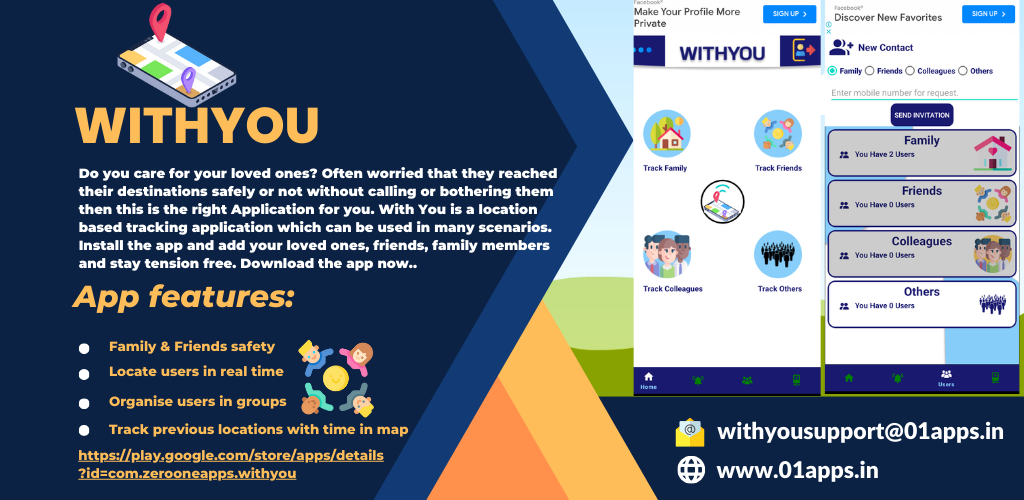