The Science
Physicists predicted the existence of the Higgs boson decades before its discovery in 2012 because of symmetries in the building blocks of our universe. Similarly, rules about a special type of symmetry called “chiral symmetry” predict the existence of subatomic particles called pions. The lifetime of a neutrally charged pion is tied to breaking of chiral symmetry. This means physicists can use theory to precisely calculate this lifetime. Measurements of this lifetime have been much less precise than calculations from theory. Now, however, physicists have measured the charge-neutral pion’s lifetime at a much higher level of precision than ever before.
The Impact
Pions are subatomic particles that carry the strong force that binds protons and neutrons together in nuclei. This force accounts for 98 percent of the mass of the visible universe. Pions are also the simplest of the subatomic particles, built of the same ingredients as protons and neutrons—quarks. As simple particles, pions are described well by the theory of quantum chromodynamics (QCD). This theory describes how our visible universe is built from the interactions of subatomic particles. Measuring the charge-neutral pion’s lifetime and comparing it to QCD thus gives insight into the particles and forces that shape our universe. With the new research, the charge-neutral pion’s measured lifetime now matches well with calculations from QCD theory.
Summary
Nuclear physicists from more than 16 collaborating U.S. and international groups formed the PrimEx collaboration to precisely measure the charge-neutral pion’s lifetime. They conducted the measurement over a series of experiments using the Continuous Electron Beam Accelerator Facility, a Department of Energy user facility. The final result yielded a measurement of roughly 83 quintillionths of a second (or 83 millionths of one trillionth of a second). This result confirms theoretical calculations from QCD with an uncertainty of just 1.50 percent. The result helps test other aspects of the theory of QCD. It also provides critical information for carrying out a precision test of the Standard Model of particle physics, the theory that describes how three of nature’s forces govern the particles that make up matter. The precision test is carried out by measuring how a particle called the muon spins in a magnetic field. The results will help physicists confirm or improve the Standard Model.
Funding
This project was supported in part by the National Science Foundation under a Major Research Instrumentation grant and by the Department of Energy Office of Science, Nuclear Physics program.
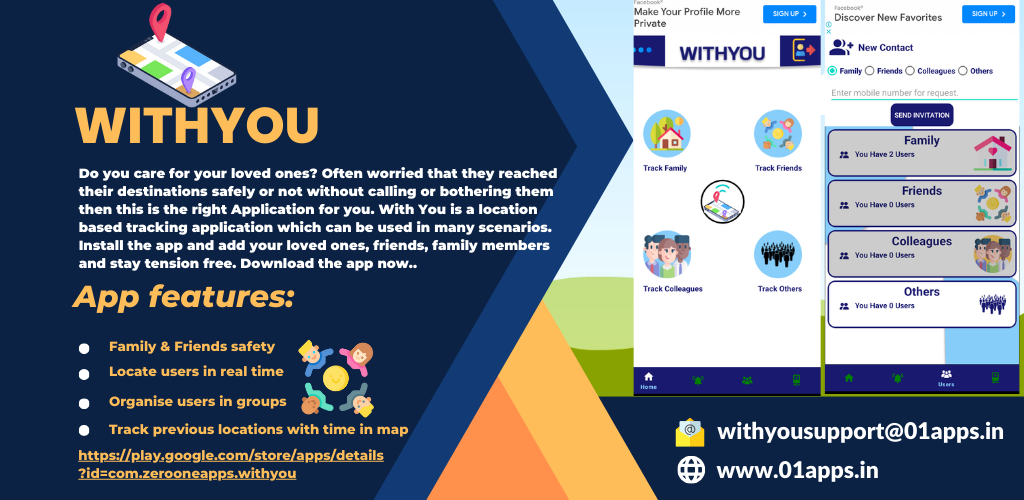