Background
BC emitted into the atmosphere by fossil fuel combustion and biomass burning efficiently absorbs solar radiation and heats the atmosphere. Furthermore, BC deposited on snow and ice can reduce their reflectivity and accelerate their melting. Most of the Arctic BC is believed to be transported from regions outside the Arctic. However, estimates of the relative contribution by a variety of sources to Arctic BC, and thus of BC’s climate impact, still have considerable uncertainties.
Research content
The research group measured vertical profiles of BC mass concentrations up to 5 km high in the Arctic in March-April 2018, during the Polar Airborne Measurements and Arctic Regional Climate Model simulation Project (PAMARCMiP) led by the Alfred Wegener Institute (AWI) in Germany. The observations were carried out using the AWI research aircraft Polar 5, and Station Nord (81.6°N, 16.7°W) as the operation base. Observed BC mass concentrations were compared with those obtained in past spring Arctic aircraft experiments (ARCTAS in 2008, HIPPO in 2010, and NETCARE in 2015) with the aim of identifying factors responsible for the year-to-year variation in BC abundance. See Figure 1.
Black carbon mass concentrations in 2018 were between 7 and 23 nanograms per cubic metre (ng m–3), which were comparable to those in 2010 (Fig.1 (left)). On the other hand, systematically higher values were observed in 2008 and 2015 at all altitudes up to 5 km. Even though each aircraft measurement was made over a limited area and time duration, these results reveal a significant year-to-year variation in BC mass concentrations in the Arctic spring.
Results
The research group found that relative changes in the year-to-year variation of “vertically integrated BC mass concentrations” – that is, the amount of BC in columns between 0 and 5 km altitudes – was generally consistent with that in biomass burning activities estimated using MODIS satellite-derived fire counts detected at latitudes north of 50ºN (Fig. 1 (right)). Transport of air influenced by biomass burnings in regions between latitudes 45–60°N and longitudes 30–50°E and 100–130°E (western and eastern Eurasia, respectively) were likely responsible for the observed increase in BC levels during the Arctic spring.
During PAMARCMiP in 2018, a pollution layer, whose sources were likely to be biomass burnings in the mid-latitudes, was occasionally visible through the windows of the research aircraft (Fig. 2). It is likely that more frequent transport of pollution from biomass burning to the Arctic had occurred during the observation periods in 2008 and 2015. See Figure 2.
The research group also investigated the extent to which current numerical model simulations can reproduce the observed year-to-year variability in BC column amounts (Fig. 1 (right)). The numerical models can separately estimate contributions from anthropogenic BC sources and that from biomass burning. The numerical models reproduced the observations relatively well in 2010 and 2018, when biomass burning activity was low, whereas they showed much smaller values than the observations in 2008 and 2015, when biomass burning activity was high. These results suggest that current numerical models generally reproduce the contribution of anthropogenic BC well, while they significantly (by a factor of three) underestimate the contribution of BC from biomass burning.
Significance of the results
Atmospheric heating effects (positive radiative forcing) of BC in the Arctic are considered to be highest in spring when BC mass concentration is highest and incoming solar radiation is increasing. BC in spring is also important because slight changes in the timing of snow/ice melt can influence the radiation budget in the Arctic. The observations presented in this study provide useful bases to improve and evaluate numerical model simulations that assess the BC radiative effect in the Arctic. Furthermore, global warming has the potential to increase biomass burning in mid- and high-latitudes. This study suggests that these future changes in BC emissions could influence the amount of Arctic BC and its radiative impacts more than estimates provided in previous studies.
The paper, “Arctic black carbon during PAMARCMiP 2018 and previous aircraft experiments in spring”, was published online in the journal “Journal: Atmospheric Chemistry and Physics” on November 4, 2021, at DOI: 10.5194/acp-21-15861-2021.
Authors:
Sho Ohata, Institute for Space–Earth Environmental Research, Nagoya University, Japan
Makoto Koike, Graduate School of Science, University of Tokyo, Japan
Atsuhi Yoshida, National Institute of Polar Research, Japan
Nobuhiro Moteki, Graduate School of Science, University of Tokyo, Japan
Kouji Adachi, Meteorological Research Institute, Japan
Naga Oshima, Meteorological Research Institute, Japan
Hitoshi Matsui, Graduate School of Environmental Studies, Nagoya University, Japan
Oliver Eppers, Johannes Gutenberg University of Mainz, Germany
Heiko Bozem, Johannes Gutenberg University of Mainz, Germany
Marco Zanatta, Université Paris-Est-Créteil, France
Andreas B. Herber, Alfred Wegener Institute Helmholtz Centre for Polar and Marine Research, Germany
Funding information
This research was partly supported by the Arctic Challenge for Sustainability (ArCS) project (JPMXD1300000000) and ArCS II (JPMXD1420318865) project of the Ministry of Education, Culture, Sports, Science, and Technology of Japan and the Environment Research and Technology Development Fund (JPMEERF20202003) of Environmental Restoration and Conservation Agency. Other funding information is found in “Acknowledgements” of the original paper.
About Nagoya University, Japan
Nagoya University has a history of about 150 years, with its roots in a temporary medical school and hospital established in 1871, and was formally instituted as the last Imperial University of Japan in 1939. Although modest in size compared to the largest universities in Japan, Nagoya University has been pursuing excellence since its founding. Six of the 18 Japanese Nobel Prize-winners since 2000 did all or part of their Nobel Prize-winning work at Nagoya University: four in Physics – Toshihide Maskawa and Makoto Kobayashi in 2008, and Isamu Akasaki and Hiroshi Amano in 2014; and two in Chemistry – Ryoji Noyori in 2001 and Osamu Shimomura in 2008. In mathematics, Shigefumi Mori did his Fields Medal-winning work at the University. A number of other important discoveries have also been made at the University, including the Okazaki DNA Fragments by Reiji and Tsuneko Okazaki in the 1960s; and depletion forces by Sho Asakura and Fumio Oosawa in 1954.
Website: https://en.nagoya-u.ac.jp/
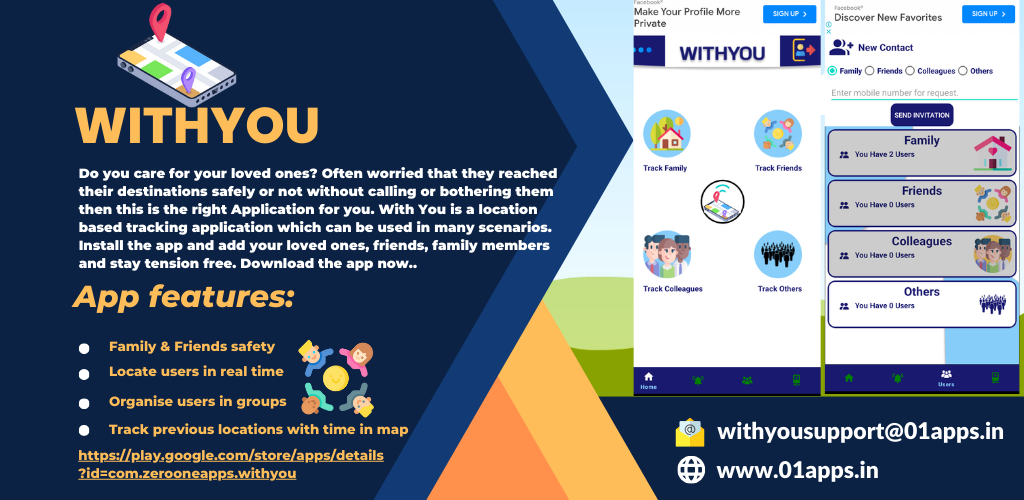